Read the full position stand on the original listing here at the ISSN Position Stand Center.
Abstract
Position Statement: The International Society of Sports Nutrition (ISSN) bases the following position stand on a critical analysis of the literature on the use of beta-hydroxy-beta-methylbutyrate (HMB) as a nutritional supplement. The ISSN has concluded the following.
1. HMB can be used to enhance recovery by attenuating exercise induced skeletal muscle damage in trained and untrained populations.
2. If consuming HMB, an athlete will benefit from consuming the supplement in close proximity to their workout.
3. HMB appears to be most effective when consumed for 2 weeks prior to an exercise bout.
4. Thirty-eight mg·kg·BM-1 daily of HMB has been demonstrated to enhance skeletal muscle hypertrophy, strength, and power in untrained and trained populations when the appropriate exercise prescription is utilized.
5. Currently, two forms of HMB have been used: Calcium HMB (HMB-Ca) and a free acid form of HMB (HMB-FA). HMB-FA may increase plasma absorption and retention of HMB to a greater extent than HMB-CA. However, research with HMB-FA is in its infancy, and there is not enough research to support whether one form is superior.
6. HMB has been demonstrated to increase LBM and functionality in elderly, sedentary populations.
7. HMB ingestion in conjunction with a structured exercise program may result in greater declines in fat mass (FM).
8. HMB’s mechanisms of action include an inhibition and increase of proteolysis and protein synthesis, respectively.
9. Chronic consumption of HMB is safe in both young and old populations.
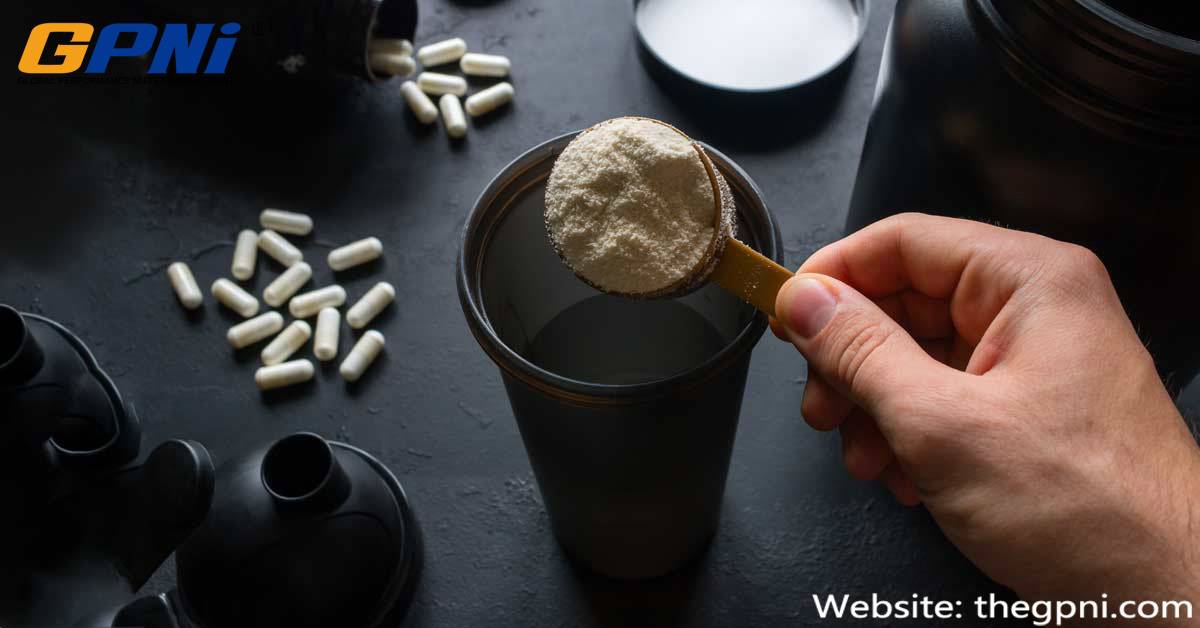
Introduction
Supplementing the diet with the amino acid leucine in combination with resistance training may increase lean body mass (LBM), strength and decrease body fat [1–3]. Moreover, leucine appears to decrease skeletal muscle soreness following eccentric exercise [4], and prevent declines in both circulating testosterone and skeletal muscle power following an overreaching cycle [5]. Leucine has been thought to augment adaptations to strength training by acting as the primary signal to activate protein synthesis (e.g. regulation of translation initiation) [1]. Additionally, for over three decades this amino acid has been known to exert antiproteolytic effects [6]. However, the effects of leucine on muscle proteolysis are maximized at 10–20 times (5–10 mM·L−1) the concentration required to maximally stimulate muscle protein synthesis [6]. Thus, it is probable that these effects are partly mediated by the conversion of leucine to a specific metabolite [7]. One strong candidate is the leucine-derived metabolite, beta-hydroxy-beta-methylbutyrate (HMB) [7, 8]. In 1996, Nissen et al. [7] first demonstrated that supplementation with HMB lowered muscle proteolysis following resistance training, and augmented gains in LBM and strength in a dose-dependent manner. Since that time HMB has been studied in a variety of anaerobic and aerobic training conditions ([9]). While numerous studies have supported the efficacy of HMB supplementation for enhancing recovery [10, 11], LBM [10, 12], strength [7], power [13], and aerobic performance [14], there have been conflicting results (Tables 1 and 2). For this reason, the primary purpose of this Position Stand is to critically analyze the existing literature on HMB supplementation and provide careful recommendations on how to optimize its effects on body composition, strength, power, and aerobic performance across varying levels of age, sex, and training status. The second purpose of this Position Stand is to critically discuss the current and proposed mechanisms of action of HMB.
Table 1 HMB effects on indices of skeletal muscle damage and breakdown
Table 2 HMB effects on body composition and performance *
Experiment
Subjects
Protocol
Periodized
Diet control
Duration/dose
Additional supplements
Body composition measures
Performance measures
Outcomes of HMB-Ca supplementation relative to placebo
Nissen 1996 [7]
Trained, NCAA football players
Monitored progressive resistance training
No
No
7 weeks, 3 grams per day HMB-Ca
No
TOBEC for total FFM and FM
Bench Press and Squat
FFM: + 1.9 % FM: - 0.5 % Strength: + 2.3 % average
Nissen 1996 [7]
Untrained college-aged males
Monitored progressive resistance training
No
Yes
3 weeks, 1.5 or 3 grams per day HMB-Ca
No
TOBEC for total FFM and FM
Strength: Average weight lifted during last 3 working sets of upper and lower body exercises
FFM: + 0.6 % FM: No Effect Strength: +2.6 to 17.4 % depending on lift
Jowko 2001 [10]
Active, college-aged males
Monitored progressive resistance training
No
No
3 weeks, 3 grams per day HMB-Ca
20 grams creatine per day for 7 days followed by 10 grams per day for 14 days
BIA
Strength: Cumulative 1-RM of major lifts (Squat, Bench Press, Clean)
FFM: + 0.6 % FM: - 0.7 % Strength: + 9 %
Kreider 1999[15]
Resistance trained, college-aged males males with > 1 year experience
Not monitored: Instructed not to change current individualized training regimens
No
No
28 days, 3 or 6 grams per day HMB-Ca
No
DXA for: LBM and FM
Strength: Bench Press and Leg Press
LBM: No Effect FM: No Effect Strength: No Effect
Gallagher 2000[12]
Untrained college-aged males
Monitored progressive resistance training
No
No
8 weeks, 3 or 6 grams per day HMB-Ca
No
7 site Skin Fold
Isometric and Isokinetic testing, Non-specific to training stimulus
FFM: + 3 % FM: - 1.6 % Strength: +2-3.5 % No differences between 3 and 6 g
Panton 2000[20]
Men and women, divided into untrained and resistance trained (> 6 months), 20–40 yrs of age
Monitored high intensity progressive resistance training
No
No
4 weeks, 3 grams per day HMB-Ca
No
Underwater Weighing
Bench Press and Leg Press 1-RM
FFM: +.5 kg FM: - .6 % Strength: +3-15 %
Hoffman 2004[19]
College Football players
Football camp, not controlled by investigators
No
No
10 days, 3 grams per day HMB-Ca
No
Not Measured
Wingate Power
No Effects
Kraemer 2009[13]
Recreationally active, college-aged males
periodized resistance training split
Yes
Yes
12 weeks, 3 grams per day HMB-Ca
14 grams arginine and 14 grams glutamine per day
DXA for LBM and FM and Limb Circumference
Squat and Bench Press 1RM Vertical Jump
LBM: + 40% FM: -40 % Strength: 50 % Power: +85 %
Thomson 2009[22]
Trained college-aged males
Non Monitored Assigned progressive resistance training program with 84 % compliance
No
No
9 weeks, 3 grams per day HMB-Ca
No
BIA
Bench Press, Preacher Curl, and Leg Extension 1-RM
FFM: 0.4 FM: - 3.8 Strength: 1.1-9.0 depending on lift
Portal 2011[23]
Elite adolescent volleyball players 13.5-18 yrs of age
Combination of progressive, resistance, and endurance exercise
Not reported
No
7 weeks, 3 grams per day HMB-Ca
No
DXA
Power on Wingate Strength of Bench Press and Leg Press
Fat: PL = +3.5% Vs. HMB= −6.6% FFM: PL= no change Vs. HMB= +3.7% Power: PL = +3% HMB = +13.5% Strength: PL=0-6.7 % vs. HMB +15.7 % - 23.5 %
Ransone 2003[24]
College football players
Progressive resistance and endurance exercise
No
No
4 weeks, 3 grams per day HMB-Ca
No
Skin Folds
Bench Press, Power Cleans, Squats 1-RM
FFM: +0.3 FM: - 3.8 Strength: 1.7 % increase
Kreider 2000 [18]
Trained, college football players
Offseason strength and conditioning program
Yes
No
4 weeks, 3 grams per day HMB-Ca
No
DXA
Bench Press, Power Cleans, Squats 1-RM, 12x6 second sprint performance
No Effects
O’Connor 2007[25]
Trained rugby players, 25 yrs of age
Progressive resistance training
No
No
6 weeks, 3 grams of HMB-Ca or HMB-Ca + Creatine per day
3 grams creatine per day
Skin Folds
Squat, Bench Press, and Deadlift 1-RM Wingate Power
Neither HMB-Ca nor creatine had an effect
Slater 2001[26]
College-aged, trained polo players and rowers
Non-controlled workouts assigned by the athletes’ respective coaches
Unknown
No
6 weeks, 3 grams per day HMB-Ca
No
DA
Bench Press, Hip Sled, Pullups 3-RM
No significant effects
HMB metabolism, pharmacokinetics and retention
Metabolism
HMB is naturally produced in animals and humans from the amino acid leucine [27]. The first step in production of HMB is the reversible transamination of leucine to α-keto-isocaproate (KIC) by the enzyme branched chain amino acid transferase [28] (Figure 1). After leucine is metabolized to KIC, KIC is either metabolized into isovaleryl-CoA by the enzymeα-ketoacid dehydrogenase in the mitochondria, or into HMB in the cytosol, by the enzymeα-ketoisocaproate dioxygenase [28]. KIC is primarily metabolized into isovaleryl-CoA, with only approximately 5% of leucine being converted into HMB [28]. To put this into perspective, an individual would need to consume over 600 g of high quality protein to obtain the amount of leucine (60 grams) necessary to produce the typical 3 g daily dosage of HMB used in human studies [9]. Since consumption of this amount of protein is impractical, HMB is typically increased via dietary supplementation.
Figure 1
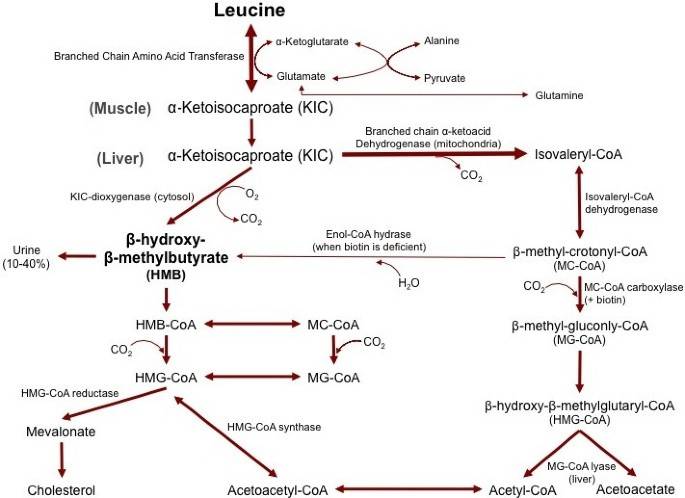
The metabolism of beta-hyroxy-beta-methyl-butyrate.
Rate of appearance and retention between varying forms of HMB
As a dietary supplement, HMB has been commercially available as a mono-hydrated calcium salt, with the empirical formula Ca (HMB)2-H2O (HMB-Ca). The magnitude and rate of appearance of HMB following ingestion is dependent on the dose, and whether or not it is consumed with additional nutrients. Specifically, Vukovich et al. [29] found that 1 g of HMB-Ca resulted in a peak HMB level in blood two hours following ingestion, while 3 g resulted in peak HMB levels 60 minutes after ingestion at 300% greater plasma concentrations (487 vs. 120 nmol·ml-1), and greater losses in urine (28% vs. 14%), for 3 and 1 g HMB-Ca ingestion, respectively. Peak HMB concentrations were also delayed by an hour and significantly lower (352 nmol·ml-1) when the HMB-Ca dosage was combined with 75 g of glucose. It is likely that the addition of glucose slowed gastric emptying, or improved HMB clearance. Recently a new delivery method of HMB, administered as a free acid, has been investigated [30]. The free acid form is called beta-hydroxy-beta-methylbutyric acid and can be designated as HMB-free acid (HMB-FA). The initial research studies have utilized HMB-FA associated with a gel, containing a buffering mechanism (K2CO3) that raises the pH to 4.5.
Commercially, HMB has only been available in the calcium salt form (HMB-Ca) as a powder, which has generally been supplemented in capsule form. Moreover, it was previously thought that because calcium dissociated relatively easily from HMB-Ca (10–15 minutes in the gut), there would be no difference in digestion kinetics between HMB-Ca and HMB-FA [31]. However, this is not the case as comparison of 0.8 g of HMB-FA to 1.0 g HMB-Ca (equivalent amounts of HMB) resulted in a doubling of peak plasma levels in one-fourth the time (30 vs. 120 minutes) in the HMB-FA compared with the HMB-Ca [30] (Figure 2). Moreover, area under the curve analysis of HMB concentrations over 180 minutes following ingestion was 91-97% greater in the HMB-FA than the HMB-Ca form. The half-life of HMB in plasma when given as HMB-FA and HMB-Ca were found to be approximately three- and two and a half hours, respectively [30]. Interestingly, even with greater peak plasma concentrations of HMB, urinary losses were not different between the two HMB forms. Perhaps the most intriguing findings were that plasma clearance, indicative of tissue uptake and utilization, was 25% greater with HMB-FA consumption compared with an equivalent HMB-CA consumption. To date, however, the majority of studies have been conducted using HMB-Ca.
Figure 2
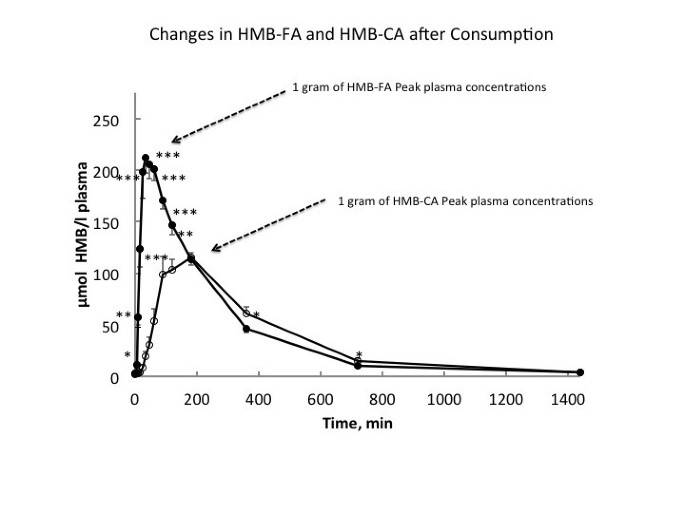
Absorbtion kinetics following ingestion of either 1 gram of calcium or free acid forms of HMB.
HMB safety
The safety of HMB has been widely studied [32–36]. In a study conducted in compliance with Food and Drug Administration Good Laboratory Practice, rats consuming a diet of up to 5% HMB-CA for 91 days did not exhibit any adverse effects vis a vis clinical observations, hematology, clinical chemistry or organ weights [36]. This study reported no observed adverse effect levels (NOAEL) of 3.49 and 4.16 g·kg·BM-1 for male and female rats, respectively [36]. This would be the equivalent of an 81 kg human male consuming almost 50 g HMB-Ca per day for three months with no adverse effects, based on human equivalent dosing (HED) normalized to body surface area. In humans, consumption of 6 g HMB·d-1 for one month had no effect on cholesterol, hemoglobin, white blood cells, blood glucose, liver or kidney function [33]. In addition, two meta-analyses, one with HMB supplementation alone and another with HMB supplementation combined with glutamine and arginine, have concluded that HMB is safe and does not result in any adverse effects [34, 35]. Moreover, Baier et al. [37] examined the effects of a 2–3 g of a daily ingestion of HMB-Ca in combination with amino acids for one year in the elderly and found that HMB consumption did not result in any changes in blood or urine markers of hepatic or renal function or blood lipids. Although the previous studies found no adverse events associated with HMB supplementation, a recent rodent study found an increase in plasma insulin after 320 mg·kg·BM-1/·d-1 supplementation for one month, which showed a significant increase in fasting insulin levels, suggesting a possible decrease in insulin sensitivity [38]. However, this finding has not been reported in any previous human study. Evidence to date indicates that that consumption of HMB is safe in both young and old populations; however, future studies examining the effects of HMB on insulin sensitivity in humans are warranted.
The effects of HMB supplementation on skeletal muscle damage, protein breakdown, and recovery
HMB is presently thought to work by speeding regenerative capacity of skeletal muscle following high intensity or prolonged exercise [7]. Researchers have used a number of dependent measures to examine this attribute including serum indices of skeletal muscle damage (creatine kinase [CK], and lactate dehydrogenase [LDH]), and urinary indicators of protein breakdown (3-methyl-histidine [3-MH] and urea nitrogen) [10, 11, 17]. Perceived recovery and skeletal muscle soreness have also been investigated following training with, and without HMB supplementation [39]. Of the studies reviewed which investigated skeletal muscle damage and recovery (Table 1), there were a variety of supplement protocols (1 day to 6 weeks; pre vs. post exercise), age ranges (19–50 yrs), training protocols (progressive resistance vs. isokinetic dynamometer), and subject-training statuses (untrained, moderately to highly resistance trained, and endurance trained). Some studies included other supplements, such as creatine monohydrate, while others consisted of HMB alone. Diet and training were controlled in some studies, but not in others (Table 1). For these reasons, results across studies have not been consistent.
Effects of training status
Training status has been a variable that has received a great deal of interest in the literature. When training and/or diet are controlled, a number of studies have demonstrated that HMB can lower indices of skeletal muscle damage and protein breakdown in a dose dependent fashion in untrained populations [7, 10, 20]. For example, Nissen et al. [7] found that HMB blunted the rise in indicators of skeletal muscle damage and protein degradation, CK, LDH, blood and urinary urea nitrogen, and 3-MH (20-60%) after three weeks of high intensity, monitored resistance exercise. However, research indicates that in trained populations it is critical that the exercise stimulus is of adequate intensity and volume to cause skeletal muscle damage [40]. If these conditions are lacking, HMB is not likely to be efficacious [9]. Kreider et al. [15] examined the effect of HMB-Ca supplementation for 28 days in resistance-trained athletes. The training protocol of this study may have affected the outcome measures of this study. Participants were instructed not to change their training intensity or volume, thus no overload throughout the duration of the study occurred. As a result, no effect of the training or HMB-Ca was observed on indices of damage. This study was the first to indicate that HMB’s effects likely interact with both the exercise stimulus and the training status of the athlete. Similarly, Kreider et al. [18] also observed no changes in muscle catabolism after 4 weeks of HMB supplementation during a 28 day offseason conditioning program in Division 1 football players. Panton et al. [20] followed up with a large cohort of 41 subjects of untrained and moderately trained subjects (> 6 months of resistance training experience). They found that HMB-Ca blunted the rise in CK levels independent of training status during a monitored, high intensity progressive resistance-training program. Knitter and colleagues [11] also found that HMB decreased skeletal muscle damage after a 20 km run in well-trained runners (> 48 km per week) as indicated by decreased CK and LDH levels after the run. Recently, Wilson et al. [41] investigated the effects of pre exercise administration of HMB-FA to resistance trained athletes on muscle damage, and perceived recovery following a high volume resistance training bout centered around squats, deadlifts, and bench press. They found that HMB-FA supplementation blunted the rise in CK levels and protein breakdown following a workout compared to the placebo group. Moreover, HMB-FA improved the perceived recovery score, suggesting that HMB-FA enhanced recovery.
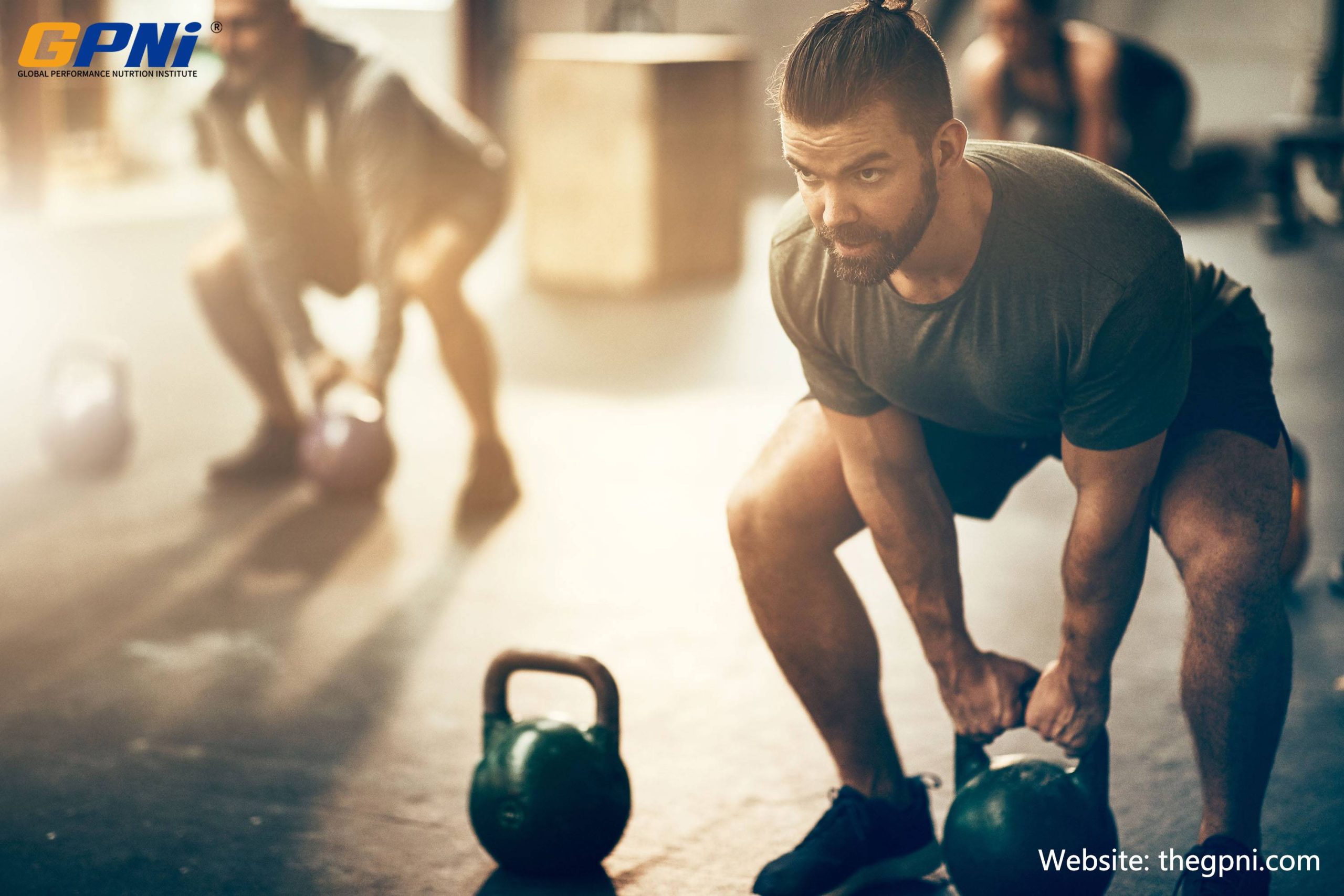
Duration of supplementation, dose, and timing
The duration, dosage, and timing of HMB supplementation have notably varied in the literature (Table 1). The first study to look at the duration and dose of HMB was conducted by Nissen and colleagues [7]. Their results indicated that HMB-Ca attenuated protein breakdown to a greater extent following two weeks of supplementation than following one week, and that HMB-Ca was not able to significantly reduce CK concentrations until the third week of training. These effects appeared to be greater when ingesting 3 g of HMB-CA compared to lower doses of the supplement (1.5 g of HMB-CA). Other investigations who have supplemented with HMB-Ca for two or more weeks have generally reported that the supplement lowers indices of skeletal muscle damage and soreness, while those supplementing for shorter periods of time have not (Table 1). These findings suggest that HMB-Ca supplementation may be optimized when ingestion begins two weeks prior to the onset of a new training period or change in training workload.
In the majority of studies, however, researchers have had subjects consume HMB-Ca with breakfast, lunch, and dinner, without any regard to how the supplement is timed relative to exercise. Currently only two studies have reported HMB’s acute effects on skeletal muscle damage and recovery. Wilson et al. [17] examined the acute and timing effects of an oral 3 g bolus of HMB-Ca supplement on 16 untrained males using a unilateral, isokinetic leg extension based training protocol. These researchers found that HMB-Ca consumed 60 minutes prior to exercise prevented a significant rise in LDH, and tended to decrease soreness of the quadriceps relative to either the HMB-Ca supplement consumed following exercise, or a placebo supplement given prior to exercise.
Collectively these findings lead us to suggest the following: HMB supplementation appears to speed recovery in untrained and trained individuals if the exercise stimulus is high intensity, and/or high volume in nature. For untrained individuals this would likely occur with the introduction of most exercise regimens; however, in a trained population the exercise stimulus will likely need to center on free weights and compound movements. In regards to optimizing HMB supplementation, it appears that HMB has both acute and chronic effects. HMB’s acute effects likely depend upon supplementation pre-exercise. If taking HMB-Ca, the recommendation would be to consume 3 g, at least 60 minutes prior to intense exercise. If consumed with glucose it may need to be taken as long as two hours prior to training. HMB in the HMB-FA form may have an overall faster and greater effect based upon the rise in plasma levels. Thus, athletes could consume the supplement in HMB-FA form 30–60 minutes prior to exercise. Finally, in order to optimize HMB’s chronic effects, the recommendation would be to consume 3 g daily, divided into three equal servings for a minimum of two weeks prior to a potentially damaging skeletal muscle event.
The effects of HMB supplementation on skeletal muscle hypertrophy in healthy untrained and trained adults
HMB’s effects on skeletal muscle mass, strength, and hypertrophy have been studied in exercising humans for nearly two decades [7, 9]. Similar to its reported effects on skeletal muscle damage, a wide range of subject populations (untrained vs. resistance trained; male vs. female) and training protocols (Table 2) have been examined. Training protocols have varied in duration (10 days to 12 weeks) [13, 19], periodization scheme [13, 42]), and training modalities (machines and free weights [22] vs. free weights only [42]) (Table 2). To confound the situation further, some researchers have designed and monitored the resistance-training protocol [7, 13, 20], while others have left it up to subjects to train on their own [15, 22]. In other cases, subjects have participated in unspecified training protocols reportedly provided by various team coaches or training camps [19, 26]. In addition, studies have provided a variety HMB doses ranging from 1.5 to 6 g daily [7, 12]. Moreover, some studies have supplemented HMB along with creatine monohydrate [10, 43] or arginine and glutamine [13]. Further, some researchers have controlled for diet [13, 42], while the majority have not [10, 12, 19, 22, 34]. Lastly, the outcome measures for indices of skeletal muscle mass have varied from less accurate indirect indices (skin fold and bioelectrical impedance measures) [10, 12, 22], to dual x-ray absorptiometry (DXA) [13] to determine fat free mass (FFM) and LBM, respectively. Thus, in order to make any overall conclusions on HMB’s effectiveness, the validity and reliability of each of these measures needs to be considered.
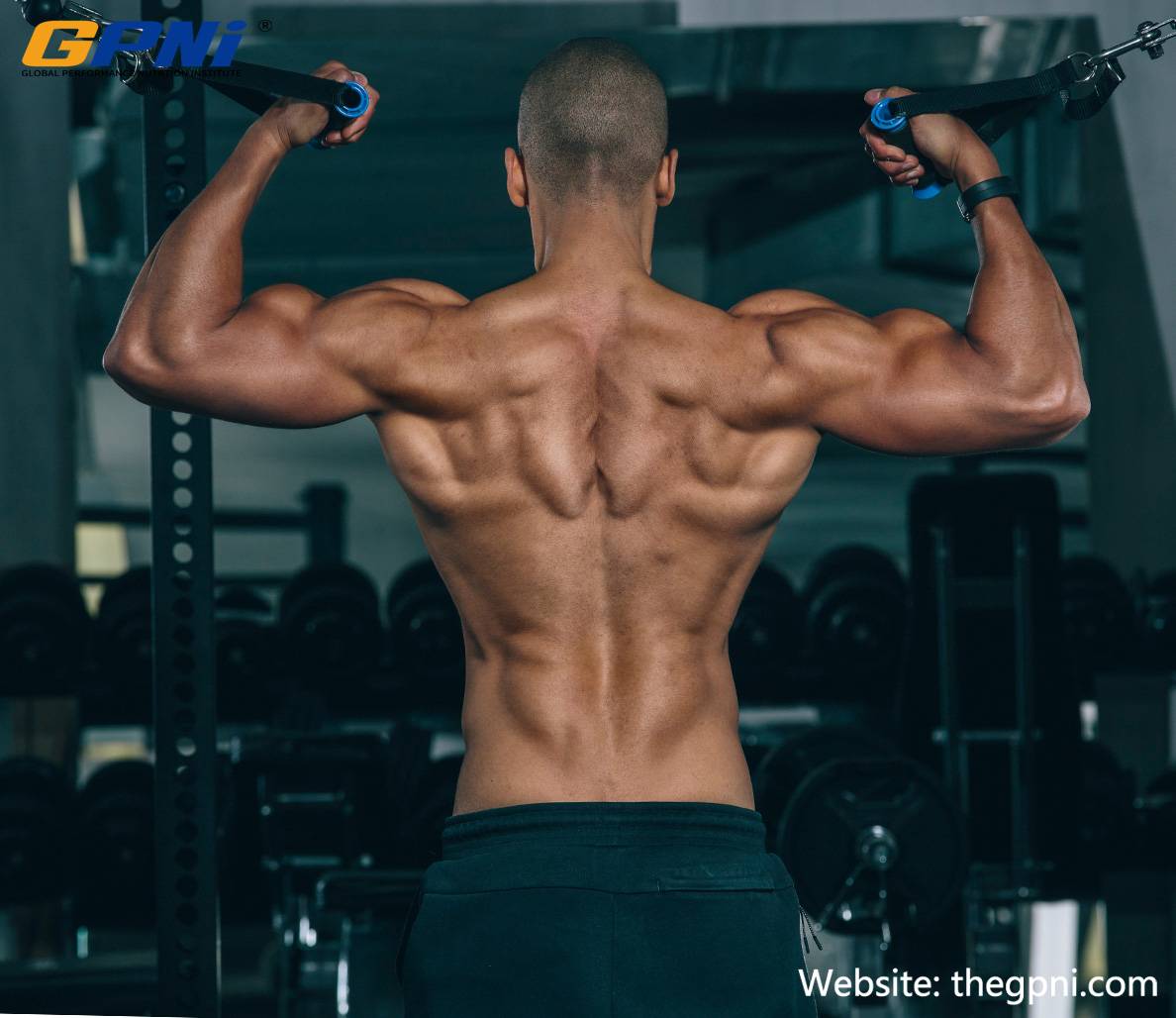
Training status and its interaction with variation of training load and duration of training protocol
Untrained individuals
In both trained and untrained individuals the majority of studies using HMB have lasted four weeks or less (Table 2). In untrained individuals supplementation with HMB has been demonstrated to increase FFM, as well as strength in as little as three weeks [7, 10]. These findings are not surprising if HMB operates through speeding recovery of damaged skeletal muscle tissue [7, 10, 20]. In particular, research indicates that the initial weeks of training result in the highest magnitude of damage in an untrained population [40, 44] (Table 2). Research supports that rate of improvement in novice lifters decline as their training experience increases, [45], however, the majority of studies using HMB were not periodized. For these reasons HMB’s magnitude of effect over a placebo in novices only slightly increases when analyzing results over eight weeks [12] versus three to four weeks utilizing a linear resistance training model [7, 10]. Finally, in untrained individuals it appears that 3 g of HMB·d-1 produces greater gains than 1.5 g of HMB·d-1[7]; though, 6 g of HMB·d-1 was not shown to further increase HMB’s effectiveness over 3 g of HMB·d-1[12]. However, only one study has examined a daily dose of 6 g HMB, therefore no definitive recommendation on (upper limit) dosing can be provided until additional research is conducted.
According to the available science, the effectiveness of HMB appears to be optimized under conditions of continually changing loading patterns [9]. Specifically, Kraemer and colleagues [13] had recreationally active, but not resistance-trained, individuals participate in a 12-week, periodized training program. Subjects were randomly assigned to 3 g daily of an HMB-Ca supplement that contained 14 g glutamine and 14 g arginine, or a placebo in a double-blinded manner. The training program consisted of three constantly changing loading patterns targeting a strength, hypertrophy, and strength endurance continuum. Moreover, these researchers controlled for subjects’ diets, and monitored every training session. Results showed that these previously untrained subjects in the HMB-Ca group experienced greater gains in LBM (+ 3.5 kg in placebo vs. + 9 kg HMB-Ca), and squat strength (+29 kg in placebo vs. + 46 kg in HMB-Ca ).
Trained individuals
The rate of adaptation in strength, power, and hypertrophy in trained and untrained individuals markedly differs. For example Ahahtanin et al. [46] found that 21 weeks of resistance training resulted in 21% and 4% increases in strength in untrained and highly strength trained athletes, respectively. In these subjects, HMB appears to augment adaptations following unaccustomed high intensity training protocols. Because the rate of adaptation is markedly slowed in trained populations it is likely that HMB’s effects in this population will be optimized over longer duration protocols (>6 weeks). For example, the majority of studies in trained individuals lasting six weeks or less found little to no significant differences with HMB-Ca compared to a placebo [15, 18, 19, 26]. However, those lasting longer than six weeks generally elicited positive effects in strength, and FFM [7, 22, 42].
The capacity of a training protocol to provide a novel training stimulus may be critical to consider when studying HMB. To date, the majority of studies have been linear in nature, and not monitored by the investigator (Table 2). The first study conducted in trained individuals lasted 28 days, and subjects were instructed to maintain their normal training protocols [15]. Neither the placebo nor HMB-Ca supplementation resulted in increases in CK or strength, thus suggesting that HMB may not work without a novel training stimulus. Following this study, Slater et al. [26] recruited trained water polo and rowing athletes. For this study the training protocol lasted six weeks, and again was not controlled by the investigators; however, the athletes were under the supervision of their respective strength coaches. As such, subdivisions of athletes in this protocol each experienced variable training stimuli making it extremely difficult to determine any direct effects of HMB supplementation. For this reason, no effects of HMB-Ca were noted.
The most recent study using HMB-Ca was conducted by Thomson and colleagues [22]. These researchers supplemented individuals with reportedly one year or more of resistance training experience with 3 g of HMB-Ca or a placebo while performing a linear (periodized) resistance-training program. Subjects were asked to follow the program for nine weeks; however, they were not monitored. Subject compliance to the training program was on average 84 ± 22%. These last two points are critical to analyze for two reasons. First, a 20% lack of compliance lowers overall training frequency, which decreases the probability of optimizing HMB’s effects on recovery rate. Second, research demonstrates that directly supervised, heavy-resistance training results in a greater rate and magnitude of training load increases in resistance-trained individuals[47]. Moreover, supervised training results in greater maximal strength gains compared with unsupervised training [48]. For this reason it is likely that the training stimulus and frequency in this nine week study did not exploit HMB’s capacity to speed recovery under maximal, and constantly varying training stimuli. An additional confounding variable in this study was that skeletal muscle hypertrophy (FFM) was estimated from bioelectrical impedance, which has been demonstrated to have high variability [49]. Finally, the outcome strength measures were single joint movements (e.g., biceps curl and leg extension). If HMB increases overall lean mass, it may have been more appropriate to select multi-joint, structural exercises such as the squat and/or bench press. However, even with these limitations nine weeks of HMB-Ca supplementation resulted in small, but statistically significant decreases in FM, and increases in FFM and strength.
To date, few studies have examined monitored resistance training in trained athletes [7, 18, 20, 42]. Of these, only one exceeded six weeks in duration. The first was conducted by Kreider et al. [18] who examined the effects of four weeks of HMB supplementation during a supervised offseason strength and conditioning program in college football players and observed no changes in lean mass or strength. However, Panton et al. [20], examined the effects of four weeks of HMB supplementation during resistance training in 36 women and 39 men (20–40 yrs) with varying levels of training experience. Their training protocol consisted of very high intensity loads (>80 % 1-RM) which were consistently adjusted as subject tolerance for a given weight increased. Due to the high intensity nature of the protocol, the HMB-Ca group showed greater decreases in body fat compared with placebo supplementation (−1.1 % vs. -0.5%, respectively); increases in bench press strength (7.5 kg vs. 5.2 kg, respectively); and LBM (1.4 kg vs.0.9 kg, respectively). These changes were independent of training experience. Moreover, Nissen et al. [7] conducted a seven week high intensity (>80% 1-RM) training study in individuals who could bench press ≥ 135 kg and squat greater than 1.5 times their bodyweight and found that subjects supplemented with HMB-Ca gained an average of 4.5 kg more on their bench press and 3.2 kg more on their squat when compared to the placebo supplemented subjects.
The most recent study using HMB-Ca was conducted by Thomson and colleagues [22]. These researchers supplemented individuals with reportedly one year or more of resistance training experience with 3 g of HMB-Ca or a placebo while performing a linear (periodized) resistance-training program. Subjects were asked to follow the program for nine weeks; however, they were not monitored. Subject compliance to the training program was on average 84 ± 22%. These last two points are critical to analyze for two reasons. First, a 20% lack of compliance lowers overall training frequency, which decreases the probability of optimizing HMB’s effects on recovery rate. Second, research demonstrates that directly supervised, heavy-resistance training results in a greater rate and magnitude of training load increases in resistance-trained individuals[47]. Moreover, supervised training results in greater maximal strength gains compared with unsupervised training [48]. For this reason it is likely that the training stimulus and frequency in this nine week study did not exploit HMB’s capacity to speed recovery under maximal, and constantly varying training stimuli. An additional confounding variable in this study was that skeletal muscle hypertrophy (FFM) was estimated from bioelectrical impedance, which has been demonstrated to have high variability [49]. Finally, the outcome strength measures were single joint movements (e.g., biceps curl and leg extension). If HMB increases overall lean mass, it may have been more appropriate to select multi-joint, structural exercises such as the squat and/or bench press. However, even with these limitations nine weeks of HMB-Ca supplementation resulted in small, but statistically significant decreases in FM, and increases in FFM and strength.
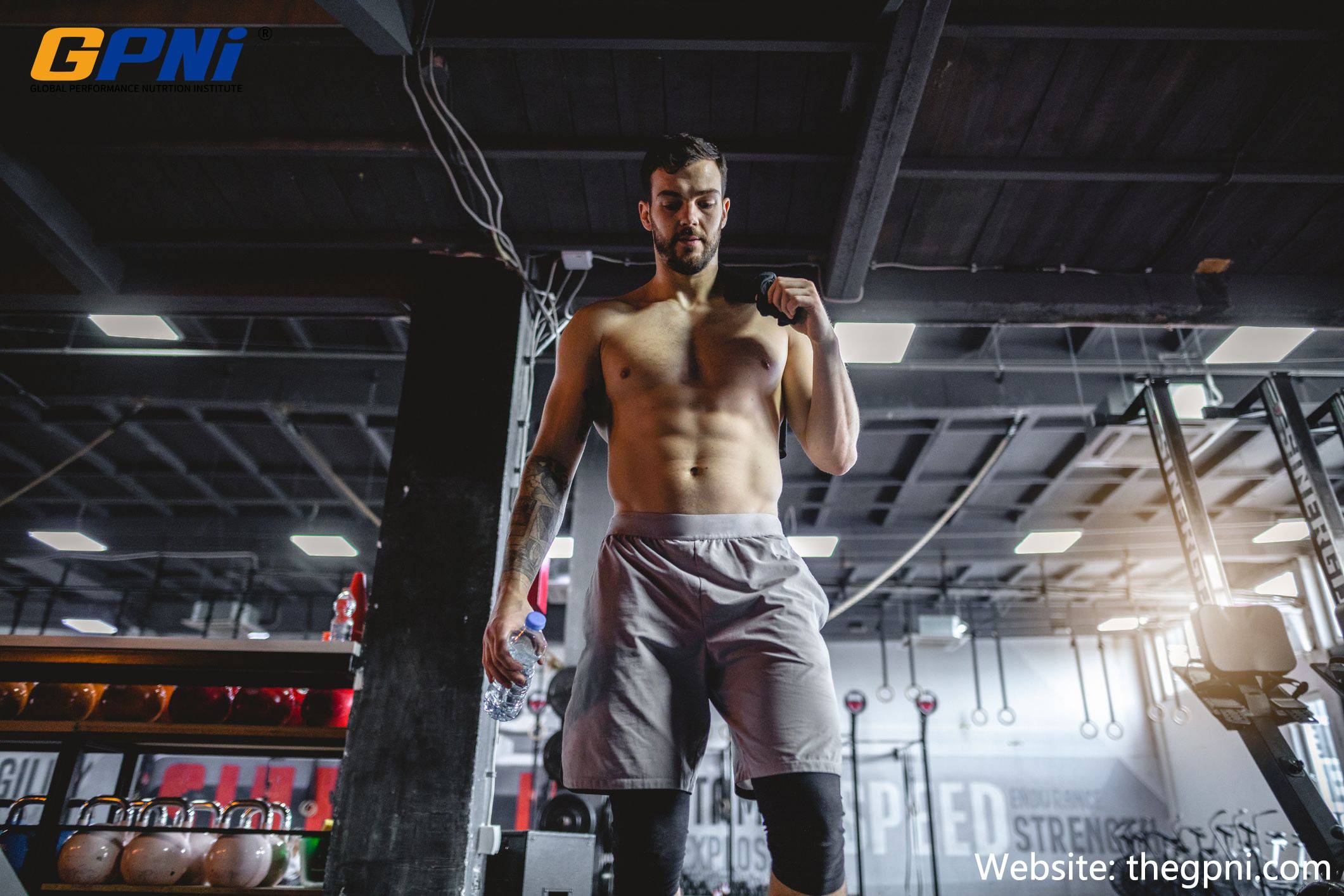
HMB in athletes training in an energy restricted state
The effects of HMB supplementation on regenerative capacity and fat metabolism make it a unique candidate for a number of special situations in which skeletal muscle wasting is indicated. One situation in particular concerns caloric (energy) restriction. Restricting calories prior to competition is commonly used by bodybuilders and those in weight-classified sports. However, research demonstrates that calorie restriction can cause decreases in lean mass and exercise performance [50]. In a recent study [50] on female judo athletes who were calorically restricted for three days, body weight and body fat percentage were significantly decreased in the subjects consuming HMB-Ca compared to the control group. There were also trends for HMB to have positive effects on LBM, which tended to decrease more in the control group (−1.6%) than in the HMB group (−0.5%). Peak power decreased by nearly 11% in the control group compared to only 5% in the HMB group. These findings suggest that individuals who are moderately calorically restricted may augment fat loss and prevent declines in LBM by supplementing with HMB.
HMB supplementation in youth and adolescent populations
Research in infants using HMB has yet to be done using human models. However, there is recent epigenetic data in animal models to suggest that HMB given during pregnancy can result in prenatal programming of skeletal muscle tissue. Specifically, maternal supplementation of HMB during pregnancy resulted in greater weight and lean mass gain in piglets than those not under maternal treatment [51]. Moreover, research in growing, pre-adolescent rats suggests that HMB supplementation was able to stimulate skeletal muscle hypertrophy in the extensor digitorum longus and soleus muscles [52], and that HMB was able to increase the mTOR and phosphorylation of p70S6K in the EDL muscle [52].
There is very little research examining the effects of HMB in human adolescent populations. However, this population may be an ideal model for HMB supplementation as resources required to augment their training adaptations compete with resources needed for normal growth of organs, bones, and muscle tissue [53–55]. HMB assists in recovery in challenging situations, and therefore may be beneficial to younger populations. In a recent study the effects of 3 grams per day of HMB-Ca on male and female elite adolescent (13–18 yrs) volleyball players during the first seven weeks of their training season was investigated [56]. Their results demonstrated that FFM increased in the HMB-Ca supplemented group, but not placebo supplemented group. Moreover FM declined (−6.6 %) in the HMB-Ca supplemented, but not placebo supplemented group (+3.5 %). In addition, Wingate test peak power, and upper- and lower-body strength were greater with HMB-Ca supplementation. No changes in hormone status (testosterone, cortisol, IGF-1, growth hormone) or inflammatory mediators (IL-6 and IL-1 receptor antagonist) occurred with HMB-Ca supplementation.
HMB supplementation in aging and masters athletes
Skeletal muscle loss is a part of the aging process and approximately 30% of skeletal muscle mass is lost between the 5th and 8th decades of life [57]. This reduction in skeletal muscle mass occurs for several reasons, including maintaining a sedentary lifestyle, malnutrition, insulin resistance, oxidative stress, and alterations in skeletal muscle metabolism and repair (as reviewed by Kim et al. [58]). In addition, the elderly exhibit impaired anabolic and anti-catabolic responsiveness to resistance exercise and amino acid feeding, termed anabolic resistance [59]. Anabolic resistance can be overcome by supplementation of leucine, and it has been hypothesized that this may be due to the conversion of leucine to HMB [52]. These data suggest a potential benefit of HMB supplementation in aging individuals [58, 60].
Studies have investigated the effects of nutritional supplements containing HMB, without an exercise intervention, on skeletal muscle mass in the elderly (reviewed by [61]). Flakoll et al. [62] investigated the effects of 12 weeks of either HMB, arginine and lysine supplementation or placebo supplementation in 50 elderly subjects and observed an increase in LBM, leg strength, handgrip strength, and a decreased “timed up and go” test time in the HMB-supplemented group compared to the placebo-supplemented group. Baier et al. [37] investigated the effects of one year of either HMB, arginine, and lysine supplementation or control supplementation in 77 elderly subjects over 65 years of age and observed significant increases in lean mass in the HMB-supplemented group and no change in lean mass in the control-supplemented group. Moreover, an increased rate of protein turnover in the HMB group and a decreased rate of protein turnover in the placebo group were observed after both three and 12 months of supplementation. In addition to the beneficial effects of HMB on skeletal muscle, HMB supplementation may also have effects on body fat. Wilson et al. [63] investigated the effects of 16 weeks of HMB supplementation in aged rats and found that body fat mass, as measured by DXA, increased by nearly 50% from young to middle age, and that HMB supplementation prevented this gain in body fat with aging. Moreover, these researchers also found that HMB supplementation was able to prevent the loss of skeletal muscle fiber size in very old as compared to young rats. These studies suggest that HMB alone can decrease body fat and increase skeletal muscle mass and strength in aging populations.
The efficacy of HMB supplementation in conjunction with a strength-training program has also been investigated in aging populations. Vukovich et al. [64] compared the effects of eight weeks of either HMB or placebo supplementation on body composition and strength in 70 year old men and women performing a strength training program. A trend (p=0.08) towards an increase in lean mass was observed in the HMB-supplemented group, while no change was observed in the placebo-supplemented group. However, it should be noted that body composition was measured with skinfold calipers in this study. The HMB-supplemented group also had an approximate 8% decrease in fat mass. Upper and lower body strength increased by 15-20%; however, there was no difference in strength changes between the groups. While the differences observed were not statistically different with HMB supplementation, it should be noted that the training protocol in this study consisted of 2 sets of 8–12 repetitions 2 days per week. Thus, this particular study suggests that in previously untrained older adults the use of HMB may not provide any further benefit than training alone. Considering the paucity of available research on HMB ingestion and resistance exercise in older adults, additional investigations are warranted.
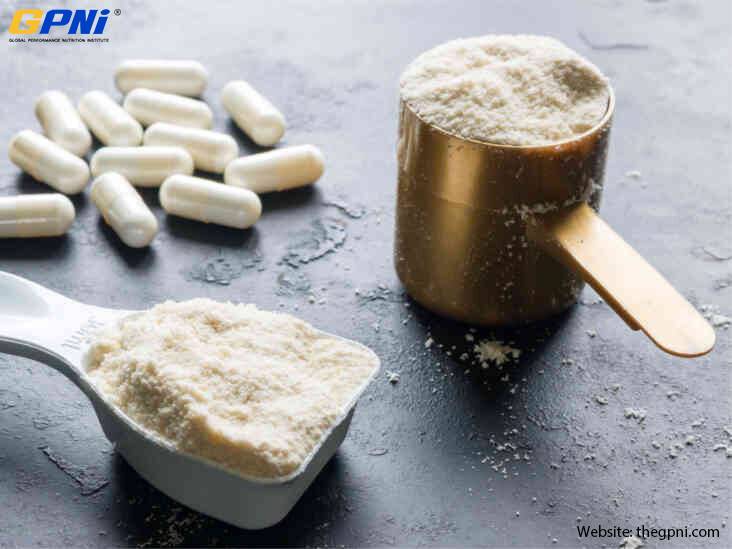
HMB improves indices of aerobic performance, fat loss, and energy metabolism
While HMB has long been touted as an anti-catabolic agent that may aid recovery and improve performance, recent evidence has identified additional metabolic benefits of HMB supplementation related to energy metabolism. This section will discuss how HMB may improve aerobic performance as well as increase fat loss and mitochondrial biogenesis, and the purported mechanisms of action underlying these benefits.
Previous studies have demonstrated the potential benefits of HMB for aerobic athletes. For instance, Vukovich and Dreifort [65] investigated the effects of HMB supplementation on peak oxygen consumption (VO2peak) and the onset of blood lactate accumulation (OBLA) in eight endurance-trained master-level competitive cyclists having an average training volume of 300 miles per week. Participants performed a graded cycle ergometer test until exhaustion. All participants performed three 2-week supplementation protocols consisting of either 3 g of HMB-Ca, 3 g of leucine, or a placebo daily, while continuing their normal training volume. Results from the graded exercise test indicated that HMB supplementation increased the time to reach VO2peak (8%), while leucine and the placebo did not. The VO2 at 2 mM blood lactate (OBLA) increased with HMB (9.1%) and leucine (2.1%) supplementation, but did not change with placebo supplementation.
The mechanisms for these benefits of HMB on aerobic performance and fat loss are poorly understood. However, recent evidence demonstrated that HMB supplementation improves fatty acid oxidation, adenosine monophosphate kinase (AMPK), Sirt1 (Silent information regulator transcripts) and Sirt3 activity in 3T3-L1 adipocytes and in skeletal muscle cells [66]. To elaborate, the Sirt proteins belong to a class of NAD+− dependent protein deacetylases involved in energy metabolism, which sense energy balance through changes in the NAD+/NADH ratio. Sirt proteins modify the acetylation level of histones and proteins [67]. Adenosine mono-phosphate protein kinase (AMPK) is also a sensor of energy balance, but does so through changes in AMP/ATP ratios [68]. Collectively, these proteins act to improve mitochondrial biogenesis, fat oxidation, energy metabolism, and the reactive oxygen defense system [67–69]. Consequently, this recent evidence has shown that HMB supplementation increases mitochondrial biogenesis and fat oxidation [70].
Exactly how HMB induces changes in Sirt proteins, AMPK, and mitochondria remains unclear. However, these results could have implications for obesity, insulin resistance, and diabetes, as well as for athletes seeking to improve body composition and aerobic performance.
Proposed mechanisms of action
Skeletal muscle protein turnover is the product of skeletal muscle protein synthesis and skeletal muscle protein degradation [71]. When protein synthesis exceeds protein degradation, there is a net synthesis of skeletal muscle protein. However, when protein degradation exceeds protein synthesis, there is a net breakdown of skeletal muscle protein. HMB has been shown to affect both protein synthesis and degradation pathways in skeletal muscle and the effect of HMB on these pathways is summarized below and in Figure 3.
Figure 3
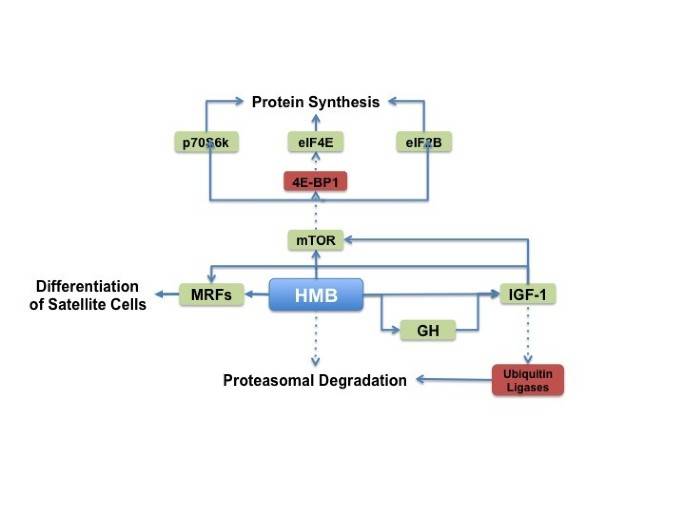
HMB’s proposed mechanisms of action.
Protein synthesis
HMB has been shown to stimulate protein synthesis in skeletal muscle [72]. This has been hypothesized to occur through stimulation of mTOR, a protein kinase responsive to mechanical, hormonal, and nutritional stimuli. Mammalian target of rapamycin has a central role in the control of cell growth, primarily by controlling mRNA translation efficiency [6]. Indeed, previous studies have observed that HMB supplementation increases phosphorylation of mTOR and its downstream targets ribosomal protein S6 kinase (S6K) and eukaryotic initiation factor-4 binding protein-1 (4EBP1) [73, 74].
The growth hormone (GH) and insulin-like growth factor 1 (IGF-1) axis may also play a key role in the stimulation of protein synthesis, and it is possible HMB may stimulate protein synthesis through changes in the activity of GH/IGF-1 axis. Gerlinger-Romero et al. [75] observed an increase in pituitary GH mRNA and protein expression after one month of HMB supplementation. Moreover, liver IGF-1 mRNA and serum IGF protein levels were also increased in the HMB-supplemented rats; however, this occurred without an increase in skeletal muscle IGF-1. In contrast, an increase in skeletal muscle insulin-like growth factor-1 (IGF-1) has been observed after HMB treatment of chicken and human myoblasts [76]. Taken together, these results suggest that HMB may affect GH/IGF-1 axis signaling; however, the effect on skeletal muscle protein synthesis requires more investigation. It is possible that the GH/IGF-1 axis signaling may require a large change in plasma HMB levels. At this point, it is not clear whether a threshold response to a specific concentration of plasma HMB exists. This certainly merits further investigation.
Skeletal muscle regeneration
The growth hormone (GH) and insulin-like growth factor 1 (IGF-1) axis may also play a key role in the stimulation of protein synthesis, and it is possible HMB may stimulate protein synthesis through changes in the activity of GH/IGF-1 axis. Gerlinger-Romero et al. [75] observed an increase in pituitary GH mRNA and protein expression after one month of HMB supplementation. Moreover, liver IGF-1 mRNA and serum IGF protein levels were also increased in the HMB-supplemented rats; however, this occurred without an increase in skeletal muscle IGF-1. In contrast, an increase in skeletal muscle insulin-like growth factor-1 (IGF-1) has been observed after HMB treatment of chicken and human myoblasts [76]. Taken together, these results suggest that HMB may affect GH/IGF-1 axis signaling; however, the effect on skeletal muscle protein synthesis requires more investigation. It is possible that the GH/IGF-1 axis signaling may require a large change in plasma HMB levels. At this point, it is not clear whether a threshold response to a specific concentration of plasma HMB exists. This certainly merits further investigation.
Skeletal muscle proteolysis
Skeletal muscle proteolysis is increased in catabolic states such as fasting, immobilization, aging, and disease [77]. HMB has been shown to decrease skeletal muscle protein degradation both in vitro[72, 73] and in vivo[78]. The mechanisms whereby HMB affects skeletal muscle protein degradation are described below.
The ubiquitin-proteasome system is an energy-dependent proteolytic system that degrades intracellular proteins. The activity of this pathway is significantly increased in conditions of exacerbated skeletal muscle catabolism, such as fasting, immobilization, bed rest and disease [77]. Therefore, inhibition of this proteolytic system could explain the attenuation of skeletal muscle protein losses observed during treatment with HMB. Indeed, HMB has been shown to decrease proteasome expression [72] and activity [72, 78–80] during catabolic states, thus attenuating skeletal muscle protein degradation through the ubiquitin-proteasome pathway.
Caspase proteases induce skeletal muscle proteolysis through apoptosis of myonuclei and are commonly up-regulated in catabolic states. However, HMB has also been shown to attenuate the up-regulation of caspases, reduce myonuclear apoptosis in catabolic states, such as skeletal muscle cells cultured with large concentrations of inflammatory cytokines [81], and skeletal muscle unloading [82]. Thus, in addition to its effects on the ubiquitin-proteasome pathway, HMB may also attenuate skeletal muscle protein degradation through inhibition of caspase activity.
Summary of mechanisms
HMB has been shown to result in a net positive balance of skeletal muscle protein turnover though stimulation of protein synthesis and attenuation of protein degradation. HMB induces protein synthesis through up-regulation of the mTOR pathway while HMB attenuates protein degradation through attenuation of the ubiquitin-proteasome pathway and caspase activity. Moreover, HMB stimulates skeletal muscle satellite cell activation and potentially increases skeletal muscle regenerative capacity.
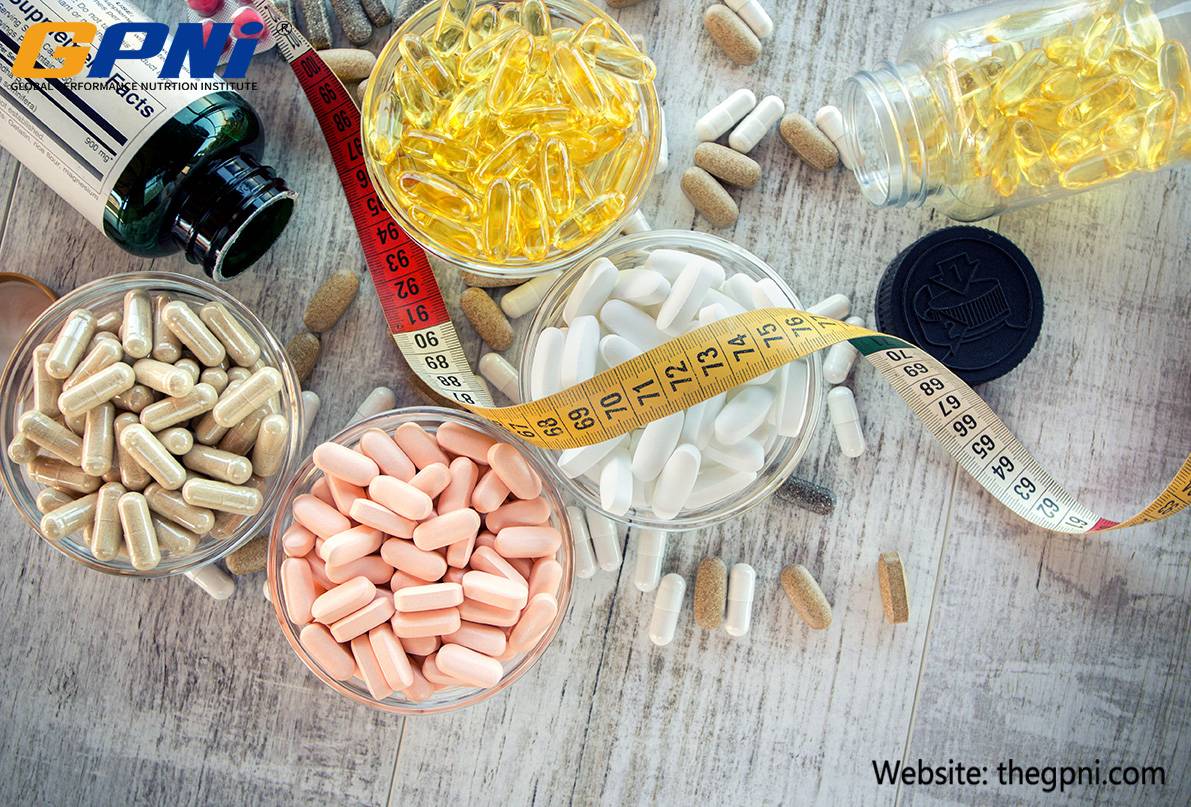
Conclusions
High intensity resistance training is essential for athletes seeking to add strength and hypertrophy. However, high intensity resistance training that results in skeletal muscle damage may take a number of days to recover from; in this case, overall training frequency may be reduced. HMB appears to speed recovery from high intensity exercise. These effects on skeletal muscle damage appear to be reliant on the timing of HMB relative to exercise, the form of HMB, the length of time HMB was supplemented prior to exercise, the dosage taken, as well as the training status of the population of interest. In particular, the supplement should be taken at 1–2 grams 30–60 minutes prior to exercise if consuming HMB-FA, and 60–120 minutes prior to exercise if consuming HMB-Ca. Finally, it is likely that HMB will work ideally if consumed at a dosage of 3 grams for two weeks prior to a high intensity bout that induces muscle damage.
HMB appears to interact with the training protocol utilized, as well as the experience of the athlete. In untrained individuals, low volume, high intensity resistance training will cause enough skeletal muscle tissue disruption to benefit from HMB supplementation. In addition to speeding recovery from high intensity exercise, HMB may assist athletes in preventing loss of lean body mass in catabolic situations such as caloric restriction. HMB may also be beneficial for augmenting body composition and physical performance in master’s level athletes, or aging individuals in general. Finally, although research is limited it appears that the supplement may also enhance aerobic performance.
References
1.
Norton LE, Layman DK: Leucine regulates translation initiation of protein synthesis in skeletal muscle after exercise. J Nutr. 2006, 136: 533S-537S.
2.
Anthony JC, Anthony TG, Layman DK: Leucine supplementation enhances skeletal muscle recovery in rats following exercise. J Nutr. 1999, 129: 1102-1106.
3.
Anthony JC, Yoshizawa F, Anthony TG, Vary TC, Jefferson LS, Kimball SR: Leucine stimulates translation initiation in skeletal muscle of postabsorptive rats via a rapamycin-sensitive pathway. J Nutr. 2000, 130: 2413-2419.
4.
Howatson G, Hoad M, Goodall S, Tallent J, Bell PG, French DN: Exercise-induced muscle damage is reduced in resistance-trained males by branched chain amino acids: a randomized, double-blind, placebo controlled study. J Int Soc Sports Nutr. 2012, 9: 20-10.1186/1550-2783-9-20.
PubMed Central CAS Article PubMed Google Scholar
5.
Kraemer WJ, Ratamess NA, Volek JS, Hakkinen K, Rubin MR, French DN, Gomez AL, McGuigan MR, Scheett TP, Newton RU, et al.: The effects of amino acid supplementation on hormonal responses to resistance training overreaching. Metabolism. 2006, 55: 282-291. 10.1016/j.metabol.2005.08.023.
CAS Article PubMed Google Scholar
6.
Zanchi NE, Nicastro H, Lancha AH: Potential antiproteolytic effects of L-leucine: observations of in vitro and in vivo studies. Nutr Metab (Lond). 2008, 5: 20-10.1186/1743-7075-5-20.
7.
Nissen S, Sharp R, Ray M, Rathmacher JA, Rice D, Fuller JC, Connelly AS, Abumrad N: Effect of leucine metabolite beta-hydroxy-beta-methylbutyrate on muscle metabolism during resistance-exercise training. J Appl Physiol. 1996, 81: 2095-2104.
8.
Nissen S, Panton L, Fuller J, Rice D, Sharp R: Effect of feeding ß-hydroxy-ß-methylbutyrate (HMB) on body composition and strength of women. FASEB J. 1997, 11: A150(abs)-
9.
Wilson GJ, Wilson JM, Manninen AH: Effects of beta-hydroxy-beta-methylbutyrate (HMB) on exercise performance and body composition across varying levels of age, sex, and training experience: a review. Nutr Metab (Lond). 2008, 5: 1-10.1186/1743-7075-5-1.
10.
Jowko E, Ostaszewski P, Jank M, Sacharuk J, Zieniewicz A, Wilczak J, Nissen S: Creatine and beta-hydroxy-beta-methylbutyrate (HMB) additively increase lean body mass and muscle strength during a weight-training program. Nutrition. 2001, 17: 558-566. 10.1016/S0899-9007(01)00540-8.
CAS Article PubMed Google Scholar
11.
Knitter AE, Panton L, Rathmacher JA, Petersen A, Sharp R: Effects of beta-hydroxy-beta-methylbutyrate on muscle damage after a prolonged run. J Appl Physiol. 2000, 89: 1340-1344.
12.
Gallagher PM, Carrithers JA, Godard MP, Schulze KE, Trappe SW: Beta-hydroxy-beta-methylbutyrate ingestion, part I: effects on strength and fat free mass. Med Sci Sports Exerc. 2000, 32: 2109-2115. 10.1097/00005768-200012000-00022.
CAS Article PubMed Google Scholar
13.
Kraemer WJ, Hatfield DL, Volek JS, Fragala MS, Vingren JL, Anderson JM, Spiering BA, Thomas GA, Ho JY, Quann EE, et al.: Effects of amino acids supplement on physiological adaptations to resistance training. Med Sci Sports Exerc. 2009, 41: 1111-1121. 10.1249/MSS.0b013e318194cc75.
CAS Article PubMed Google Scholar
14.
Vukovich M, Dreifort G: Effect of β-Hydroxy β-Methylbutyrate on the Onset of Blood Lactate Accumulation and O2peak in Endurance-Trained Cyclists. J Strength Cond Res. 2001, 15: 491-497.
15.
Kreider RB, Ferreira M, Wilson M, Almada AL: Effects of calcium beta-hydroxy-beta-methylbutyrate (HMB) supplementation during resistance-training on markers of catabolism, body composition and strength. Int J Sports Med. 1999, 20: 503-509. 10.1055/s-1999-8835.
CAS Article PubMed Google Scholar
16.
Paddon-Jones D, Keech A, Jenkins D: Short-term beta-hydroxy-beta-methylbutyrate supplementation does not reduce symptoms of eccentric muscle damage. Int J Sport Nutr Exerc Metab. 2001, 11: 442-450.
17.
Wilson JM, Kim JS, Lee SR, Rathmacher JA, Dalmau B, Kingsley JD, Koch H, Manninen AH, Saadat R, Panton LB: Acute and timing effects of beta-hydroxy-beta-methylbutyrate (HMB) on indirect markers of skeletal muscle damage. Nutr Metab. 2009, 6: 6-10.1186/1743-7075-6-6.
18.
Kreider RB, Ferreira M, Greenwood M, Wilson M, Grindstaff P, Plisk J, Reinardy E, Cantler E, Almada AL: Effects of Calcium B-HMB supplementation during training on markers of body composition, strength, and sprint performance. J Exerc Physiology-online. 2000, 3: 48-59.
19.
Hoffman JR, Cooper J, Wendell M, Im J, Kang J: Effects of beta-hydroxy beta-methylbutyrate on power performance and indices of muscle damage and stress during high-intensity training. J Strength Conditioning Res/ National Strength & Conditioning Assoc. 2004, 18: 747-752.
20.
Panton LB, Rathmacher JA, Baier S, Nissen S: Nutritional supplementation of the leucine metabolite beta-hydroxy-beta-methylbutyrate (hmb) during resistance training. Nutrition. 2000, 16: 734-739. 10.1016/S0899-9007(00)00376-2.
CAS Article PubMed Google Scholar
21.
van Someren KA, Edwards AJ, Howatson G: Supplementation with beta-hydroxy-beta-methylbutyrate (HMB) and alpha-ketoisocaproic acid (KIC) reduces signs and symptoms of exercise-induced muscle damage in man. Int J Sport Nutr Exerc Metab. 2005, 15: 413-424.
22.
Thomson JS, Watson PE, Rowlands DS: Effects of nine weeks of beta-hydroxy-beta- methylbutyrate supplementation on strength and body composition in resistance trained men. J Strength Conditioning Res/ National Strength & Conditioning Assoc. 2009, 23: 827-835. 10.1519/JSC.0b013e3181a00d47.
23.
Portal S, Zadik Z, Rabinowitz J, Pilz-Burstein R, Adler-Portal D, Meckel Y, Cooper DM, Eliakim A, Nemet D: The effect of HMB supplementation on body composition, fitness, hormonal and inflammatory mediators in elite adolescent volleyball players: a prospective randomized, double-blind, placebo-controlled study. Eur J Appl Physiol. 2011, 111: 2261-2269. 10.1007/s00421-011-1855-x.
CAS Article PubMed Google Scholar
24.
Ransone J, Neighbors K, Lefavi R, Chromiak J: The effect of beta-hydroxy beta-methylbutyrate on muscular strength and body composition in collegiate football players. J Strength Cond Res. 2003, 17: 34-39.
25.
O’Connor DM, Crowe MJ: Effects of six weeks of beta-hydroxy-beta-methylbutyrate (HMB) and HMB/creatine supplementation on strength, power, and anthropometry of highly trained athletes. J Strength Conditioning Res/ National Strength & Conditioning Assoc. 2007, 21: 419-423.
26.
Slater G, Jenkins D, Logan P, Lee H, Vukovich M, Rathmacher JA, Hahn AG: Beta-hydroxy-beta-methylbutyrate (HMB) supplementation does not affect changes in strength or body composition during resistance training in trained men. Int J Sport Nutr Exerc Metab. 2001, 11: 384-396.
27.
Van Koevering MT, Dolezal HG, Gill DR, Owens FN, Strasia CA, Buchanan DS, Lake R, Nissen S: Effects of beta-hydroxy-beta-methyl butyrate on performance and carcass quality of feedlot steers. J Anim Sci. 1994, 72: 1927-1935.
28.
Zanchi NE, Gerlinger-Romero F, Guimaraes-Ferreira L, de Siqueira Filho MA, Felitti V, Lira FS, Seelaender M, Lancha AH: HMB supplementation: clinical and athletic performance-related effects and mechanisms of action. Amino Acids. 2011, 40: 1015-1025. 10.1007/s00726-010-0678-0.
CAS Article PubMed Google Scholar
29.
Vukovich MD, Slater G, Macchi MB, Turner MJ, Fallon K, Boston T, Rathmacher J: beta-hydroxy-beta-methylbutyrate (HMB) kinetics and the influence of glucose ingestion in humans. J Nutr Biochem. 2001, 12: 631-639. 10.1016/S0955-2863(01)00182-6.
CAS Article PubMed Google Scholar
30.
Fuller JC, Sharp RL, Angus HF, Baier SM, Rathmacher JA: Free acid gel form of beta-hydroxy-beta-methylbutyrate (HMB) improves HMB clearance from plasma in human subjects compared with the calcium HMB salt. Br J Nutr. 2011, 105: 367-372. 10.1017/S0007114510003582.
CAS Article PubMed Google Scholar
31.
Baxter J, Phillips R, Dowlati L, Goehring K, Johns P: Direct Determination of Beta-Hydroxy-Beta-Methylbutyrate (HMB) in Liquid Nutrition Products. Food Analytical Methods. 2011, 4: 341-346. 10.1007/s12161-010-9173-1.
32.
Nissen SL, Abumrad NN: Nutritional role of the leucine metabolite B-hydroxy B-methylbutyrate (HMB). J Nutr Biochem. 1997, 8: 300-311. 10.1016/S0955-2863(97)00048-X.
33.
Gallagher PM, Carrithers JA, Godard MP, Schulze KE, Trappe SW: Beta-hydroxy-beta-methylbutyrate ingestion, part II: effects on hematology, hepatic and renal function. Med Sci Sports Exerc. 2000, 32: 2116-2119. 10.1097/00005768-200012000-00023.
CAS Article PubMed Google Scholar
34.
Nissen S, Sharp RL, Panton L, Vukovich M, Trappe S, Fuller JC: beta-hydroxy-beta-methylbutyrate (HMB) supplementation in humans is safe and may decrease cardiovascular risk factors. J Nutr. 2000, 130: 1937-1945.
35.
Rathmacher JA, Nissen S, Panton L, Clark RH, Eubanks May P, Barber AE, D’Olimpio J, Abumrad NN: Supplementation with a combination of beta-hydroxy-beta-methylbutyrate (HMB), arginine, and glutamine is safe and could improve hematological parameters. JPEN J Parenter Enteral Nutr. 2004, 28: 65-75. 10.1177/014860710402800265.
CAS Article PubMed Google Scholar
36.
Baxter JH, Carlos JL, Thurmond J, Rehani RN, Bultman J, Frost D: Dietary toxicity of calcium beta-hydroxy-beta-methyl butyrate (CaHMB). Food Chem Toxicol. 2005, 43: 1731-1741. 10.1016/j.fct.2005.05.016.
CAS Article PubMed Google Scholar
37.
Baier S, Johannsen D, Abumrad N, Rathmacher JA, Nissen S, Flakoll P: Year-long changes in protein metabolism in elderly men and women supplemented with a nutrition cocktail of beta-hydroxy-beta-methylbutyrate (HMB), L-arginine, and L-lysine. JPEN J Parenteral Enteral Nutr. 2009, 33: 71-82.
38.
da Justa Pinheiro CH, et al.: Metabolic and functional effects of beta-hydroxy-beta-methylbutyrate (HMB) supplementation in skeletal muscle. Eur J Appl Physiol. 2012, 112: 2531-2537. 10.1007/s00421-011-2224-5.
39.
Sikorski EM, Wilson JM, Lowery RP, Duncan NM, Davis GS, Rathmacher JA, Baier S, Naimo MA, Wilson SMC, Dunsmore KA, et al.: The acute effects of a free acid beta-hydoxy-beta-methyl butyrate supplement on muscle damage following resistance training: a randomized, double-blind, placebo-controlled study. J Int Soc Sports Nutr. 2012, 9 (Suppl 1): 27-
40.
Clarkson PM, Hubal MJ: Exercise-induced muscle damage in humans. Am J Phys Med Rehabil. 2002, 81: S52-S69. 10.1097/00002060-200211001-00007.
41.
Wilson JM, Lowery RP, Joy JM, Walters JA, Baier SM, Fuller JC, Stout JR, Norton LE, Sikorski EM, Wilson SM, et al.: beta-Hydroxy-beta-methylbutyrate free acid reduces markers of exercise-induced muscle damage and improves recovery in resistance-trained men. Br J Nutr. 2013, 3: 1-7. Epub ahead of print
42.
Dunsmore KA, Lowery RP, Duncan NM, Davis GS, Rathmacher JA, Baier SM, Sikorski EM, Morrison TJ, Naimo MA, Walters J, et al.: Effects of 12 weeks of beta-hydroxy-beta-methylbutyrate free acid Gel supplementation on muscle mass, strength, and power in resistance trained individuals. J Int Soc Sports Nutr. 2012, 9 (Suppl 1): 5-
43.
O’Connor DM, Crowe MJ: Effects of six weeks of beta-hydroxy-beta-methylbutyrate (HMB) and HMB/creatine supplementation on strength, power, and anthropometry of highly trained athletes. J Strength Cond Res. 2007, 21: 419-423.
44.
McHugh MP, Connolly DA, Eston RG, Gleim GW: Exercise-induced muscle damage and potential mechanisms for the repeated bout effect. Sports Med. 1999, 27: 157-170. 10.2165/00007256-199927030-00002.
CAS Article PubMed Google Scholar
45.
Turner A: The science and practice of periodization: a brief review. Strength Conditioning J. 2011, 33:.
46.
Ahtiainen JP, Pakarinen A, Alen M, Kraemer WJ, Hakkinen K: Muscle hypertrophy, hormonal adaptations and strength development during strength training in strength-trained and untrained men. Eur J Appl Physiol. 2003, 89: 555-563. 10.1007/s00421-003-0833-3.
CAS Article PubMed Google Scholar
47.
Mazzetti SA, Kraemer WJ, Volek JS, Duncan ND, Ratamess NA, Gomez AL, Newton RU, Hakkinen K, Fleck SJ: The influence of direct supervision of resistance training on strength performance. Med Sci Sports Exerc. 2000, 32: 1175-1184. 10.1097/00005768-200006000-00023.
CAS Article PubMed Google Scholar
48.
Ratamess NA, Faigenbaum AD, Hoffman JR, Kang J: Self-selected resistance training intensity in healthy women: the influence of a personal trainer. J Strength Conditioning Res/ National Strength & Conditioning Assoc. 2008, 22: 103-111. 10.1519/JSC.0b013e31815f29cc.
49.
Matthie JR: Bioimpedance measurements of human body composition: critical analysis and outlook. Expert Rev Med Devices. 2008, 5: 239-261. 10.1586/17434440.5.2.239.
50.
Hunga W, Liub T-H, Chenc C-Y, Chang C-K: Effect of [beta]-hydroxy-[beta]-methylbutyrate Supplementation During Energy Restriction in Female Judo Athletes. J Exerc Sci Fitness. 2010, 8: 50-53. 10.1016/S1728-869X(10)60007-X.
51.
Tatara MR, Krupski W, Tymczyna B, Studzinski T: Effects of combined maternal administration with alpha-ketoglutarate (AKG) and beta-hydroxy-beta-methylbutyrate (HMB) on prenatal programming of skeletal properties in the offspring. Nutr Metab (Lond). 2012, 9: 39-10.1186/1743-7075-9-39.
52.
Pimentel GD, Rosa JC, Lira FS, Zanchi NE, Ropelle ER, Oyama LM, Nascimento CM Od, de Mello MT, Tufik S, Santos RV: beta-Hydroxy-beta-methylbutyrate (HMbeta) supplementation stimulates skeletal muscle hypertrophy in rats via the mTOR pathway. Nutr Metab. 2011, 8: 11-10.1186/1743-7075-8-11.
53.
Goran MI: Energy expenditure, body composition, and disease risk in children and adolescents. Proc Nutr Soc. 1997, 56: 195-209. 10.1079/PNS19970025.
CAS Article PubMed Google Scholar
54.
Goran MI, Sun M: Total energy expenditure and physical activity in prepubertal children: recent advances based on the application of the doubly labeled water method. Am J Clin Nutr. 1998, 68: 944S-949S.
55.
Hartman JW, Tang JE, Wilkinson SB, Tarnopolsky MA, Lawrence RL, Fullerton AV, Phillips SM: Consumption of fat-free fluid milk after resistance exercise promotes greater lean mass accretion than does consumption of soy or carbohydrate in young, novice, male weightlifters. Am J Clin Nutr. 2007, 86: 373-381.
56.
Zadik Z, Nemet D, Eliakim A: “Hormonal and metabolic effects of nutrition in athletes”. J Pediatr Endocrinol Metab. 2009, 22 (9): 769-778.
57.
Larsson L, Grimby G, Karlsson J: Muscle strength and speed of movement in relation to age and muscle morphology. J Appl Physiol. 1979, 46: 451-456.
58.
Kim JS, Wilson JM, Lee SR: Dietary implications on mechanisms of sarcopenia: roles of protein, amino acids and antioxidants. J Nutr Biochem. 2010, 21: 1-13. 10.1016/j.jnutbio.2009.06.014.
59.
Fry CS, Rasmussen BB: Skeletal muscle protein balance and metabolism in the elderly. Curr Aging Sci. 2011, 4: 260-268. 10.2174/1874609811104030260.
CAS Article PubMed Google Scholar
60.
Katsanos CS, Kobayashi H, Sheffield-Moore M, Aarsland A, Wolfe RR: A high proportion of leucine is required for optimal stimulation of the rate of muscle protein synthesis by essential amino acids in the elderly. Am J Physiol Endocrinol Metab. 2006, 291: E381-E387. 10.1152/ajpendo.00488.2005.
CAS Article PubMed Google Scholar
61.
Fitschen PJ, Wilson GJ, Wilson JM, Wilund KR: Efficacy of beta-hydroxy-beta-methylbutyrate supplementation in elderly and clinical populations. Nutrition. 2013, 29 (1): 29-36. 10.1016/j.nut.2012.05.005.
CAS Article PubMed Google Scholar
62.
Flakoll P, Sharp R, Baier S, Levenhagen D, Carr C, Nissen S: Effect of beta-hydroxy-beta-methylbutyrate, arginine, and lysine supplementation on strength, functionality, body composition, and protein metabolism in elderly women. Nutrition. 2004, 20: 445-451. 10.1016/j.nut.2004.01.009.
CAS Article PubMed Google Scholar
63.
Wilson JM, Grant SC, Lee SR, Masad IS, Park YM, Henning PC, Stout JR, Loenneke JP, Arjmandi BH, Panton LB, Kim JS: Beta-hydroxy-beta-methyl-butyrate blunts negative age-related changes in body composition, functionality and myofiber dimensions in rats. J Int Soc Sports Nutr. 2012, 9: 18-10.1186/1550-2783-9-18.
PubMed Central CAS Article PubMed Google Scholar
64.
Vukovich MD, Stubbs NB, Bohlken RM: Body composition in 70-year-old adults responds to dietary beta-hydroxy-beta-methylbutyrate similarly to that of young adults. J Nutr. 2001, 131: 2049-2052.
65.
Vukovich MD, Dreifort GD: Effect of beta-hydroxy beta-methylbutyrate on the onset of blood lactate accumulation and V(O)(2) peak in endurance-trained cyclists. J Strength Conditioning Res/ National Strength & Conditioning Assoc. 2001, 15: 491-497.
66.
Bruckbauer A, Zemel MB, Thorpe T, Akula MR, Stuckey AC, Osborne D, Martin EB, Kennel S, Wall JS: Synergistic effects of leucine and resveratrol on insulin sensitivity and fat metabolism in adipocytes and mice. Nutr Metab (Lond). 2012, 9: 77-10.1186/1743-7075-9-77.
67.
Verdin E, Hirschey MD, Finley LW, Haigis MC: Sirtuin regulation of mitochondria: energy production, apoptosis, and signaling. Trends Biochem Sci. 2010, 35: 669-675. 10.1016/j.tibs.2010.07.003.
PubMed Central CAS Article PubMed Google Scholar
68.
Hardie DG: Minireview: the AMP-activated protein kinase cascade: the key sensor of cellular energy status. Endocrinology. 2003, 144: 5179-5183. 10.1210/en.2003-0982.
CAS Article PubMed Google Scholar
69.
Hardie DG, Hawley SA, Scott JW: AMP-activated protein kinase–development of the energy sensor concept. J Physiol. 2006, 574: 7-15. 10.1113/jphysiol.2006.108944.
PubMed Central CAS Article PubMed Google Scholar
70.
Zemel RASMB: Role of β-hydroxy-β-methylbutyrate (HMB) in leucine stimulation of muscle mitochondrial biogenesis. FASEB J. 2012, 26: 251.6-
71.
Wilson GJ, Layman DK, Moulton CJ, Norton LE, Anthony TG, Proud CG, Rupassara SI, Garlick PJ: Leucine or carbohydrate supplementation reduces AMPK and eEF2 phosphorylation and extends postprandial muscle protein synthesis and rats. Am J Physiol Endocrinol Metab. 2011, 301 (6): E1236-E1242. 10.1152/ajpendo.00242.2011.
PubMed Central CAS Article PubMed Google Scholar
72.
Smith HJ, Mukerji P, Tisdale MJ: Attenuation of proteasome-induced proteolysis in skeletal muscle by {beta}-hydroxy-{beta}-methylbutyrate in cancer-induced muscle loss. Cancer Res. 2005, 65: 277-283.
CAS Article PubMed Google Scholar
73.
Eley HL, Russell ST, Baxter JH, Mukerji P, Tisdale MJ: Signaling pathways initiated by beta-hydroxy-beta-methylbutyrate to attenuate the depression of protein synthesis in skeletal muscle in response to cachectic stimuli. Am J Physiol Endocrinol Metab. 2007, 293: E923-E931. 10.1152/ajpendo.00314.2007.
CAS Article PubMed Google Scholar
74.
Aversa Z, Bonetto A, Costelli P, Minero VG, Penna F, Baccino FM, Lucia S, Rossi Fanelli F, Muscaritoli M: beta-hydroxy-beta-methylbutyrate (HMB) attenuates muscle and body weight loss in experimental cancer cachexia. Int J Oncol. 2011, 38: 713-720.
75.
Gerlinger-Romero F, Guimaraes-Ferreira L, Giannocco G, Nunes MT: Chronic supplementation of beta-hydroxy-beta methylbutyrate (HMbeta) increases the activity of the GH/IGF-I axis and induces hyperinsulinemia in rats. Growth hormone & IGF research: official journal of the Growth Hormone Research Society and the International IGF Research Society. 2011, 21: 57-62. 10.1016/j.ghir.2010.12.006.
76.
Kornasio R, Riederer I, Butler-Browne G, Mouly V, Uni Z, Halevy O: Beta-hydroxy-beta-methylbutyrate (HMB) stimulates myogenic cell proliferation, differentiation and survival via the MAPK/ERK and PI3K/Akt pathways. Biochim Biophys Acta. 2009, 1793: 755-763. 10.1016/j.bbamcr.2008.12.017.
CAS Article PubMed Google Scholar
77.
Lecker SH, Jagoe RT, Gilbert A, Gomes M, Baracos V, Bailey J, Price SR, Mitch WE, Goldberg AL: Multiple types of skeletal muscle atrophy involve a common program of changes in gene expression. FASEB J. 2004, 18: 39-51. 10.1096/fj.03-0610com.
CAS Article PubMed Google Scholar
78.
Holecek M, Muthny T, Kovarik M, Sispera L: Effect of beta-hydroxy-beta-methylbutyrate (HMB) on protein metabolism in whole body and in selected tissues. Food and chemical toxicology: an international journal published for the British Industrial Biological Research Association. 2009, 47: 255-259. 10.1016/j.fct.2008.11.021.
79.
Kovarik M, Muthny T, Sispera L, Holecek M: Effects of beta-hydroxy-beta-methylbutyrate treatment in different types of skeletal muscle of intact and septic rats. J Physiol Biochem. 2010, 66: 311-319. 10.1007/s13105-010-0037-3.
CAS Article PubMed Google Scholar
80.
Smith HJ, Wyke SM, Tisdale MJ: Mechanism of the attenuation of proteolysis-inducing factor stimulated protein degradation in muscle by beta-hydroxy-beta-methylbutyrate. Cancer Res. 2004, 64: 8731-8735. 10.1158/0008-5472.CAN-04-1760.
CAS Article PubMed Google Scholar
81.
Eley HL, Russell ST, Tisdale MJ: Mechanism of attenuation of muscle protein degradation induced by tumor necrosis factor-alpha and angiotensin II by beta-hydroxy-beta-methylbutyrate. Am J Physiol Endocrinol Metab. 2008, 295: E1417-E1426. 10.1152/ajpendo.90567.2008.
CAS Article PubMed Google Scholar
82.
Hao Y, Jackson JR, Wang Y, Edens N, Pereira SL, Alway SE: Am J Physiol Regul Integr Comp Physiol. 2011, 301: R701-R715. 10.1152/ajpregu.00840.2010.