Read the full position stand on the original listing here at the ISSN Position Stand Center.
Abstract
Position statement: The International Society of Sports Nutrition (ISSN) provides an objective and critical review of the mechanisms and use of probiotic supplementation to optimize the health, performance, and recovery of athletes. Based on the current available literature, the conclusions of the ISSN are as follows:
1、Probiotics are live microorganisms that, when administered in adequate amounts, confer a health benefit on the host (FAO/WHO).
2、Probiotic administration has been linked to a multitude of health benefits, with gut and immune health being the most researched applications.
3、Despite the existence of shared, core mechanisms for probiotic function, health benefits of probiotics are strain- and dose-dependent.
4、Athletes have varying gut microbiota compositions that appear to reflect the activity level of the host in comparison to sedentary people, with the differences linked primarily to the volume of exercise and amount of protein consumption. Whether differences in gut microbiota composition affect probiotic efficacy is unknown.
5、The main function of the gut is to digest food and absorb nutrients. In athletic populations, certain probiotics strains can increase absorption of key nutrients such as amino acids from protein, and affect the pharmacology and physiological properties of multiple food components.
6、Immune depression in athletes worsens with excessive training load, psychological stress, disturbed sleep, and environmental extremes, all of which can contribute to an increased risk of respiratory tract infections. In certain situations, including exposure to crowds, foreign travel and poor hygiene at home, and training or competition venues, athletes’ exposure to pathogens may be elevated leading to increased rates of infections. Approximately 70% of the immune system is located in the gut and probiotic supplementation has been shown to promote a healthy immune response. In an athletic population, specific probiotic strains can reduce the number of episodes, severity and duration of upper respiratory tract infections.
7、Intense, prolonged exercise, especially in the heat, has been shown to increase gut permeability which potentially can result in systemic toxemia. Specific probiotic strains can improve the integrity of the gut-barrier function in athletes.
8、Administration of selected anti-inflammatory probiotic strains have been linked to improved recovery from muscle-damaging exercise.
9、The minimal effective dose and method of administration (potency per serving, single vs. split dose, delivery form) of a specific probiotic strain depends on validation studies for this particular strain. Products that contain probiotics must include the genus, species, and strain of each live microorganism on its label as well as the total estimated quantity of each probiotic strain at the end of the product’s shelf life, as measured by colony forming units (CFU) or live cells.
10、Preclinical and early human research has shown potential probiotic benefits relevant to an athletic population that include improved body composition and lean body mass, normalizing age-related declines in testosterone levels, reductions in cortisol levels indicating improved responses to a physical or mental stressor, reduction of exercise-induced lactate, and increased neurotransmitter synthesis, cognition and mood. However, these potential benefits require validation in more rigorous human studies and in an athletic population.
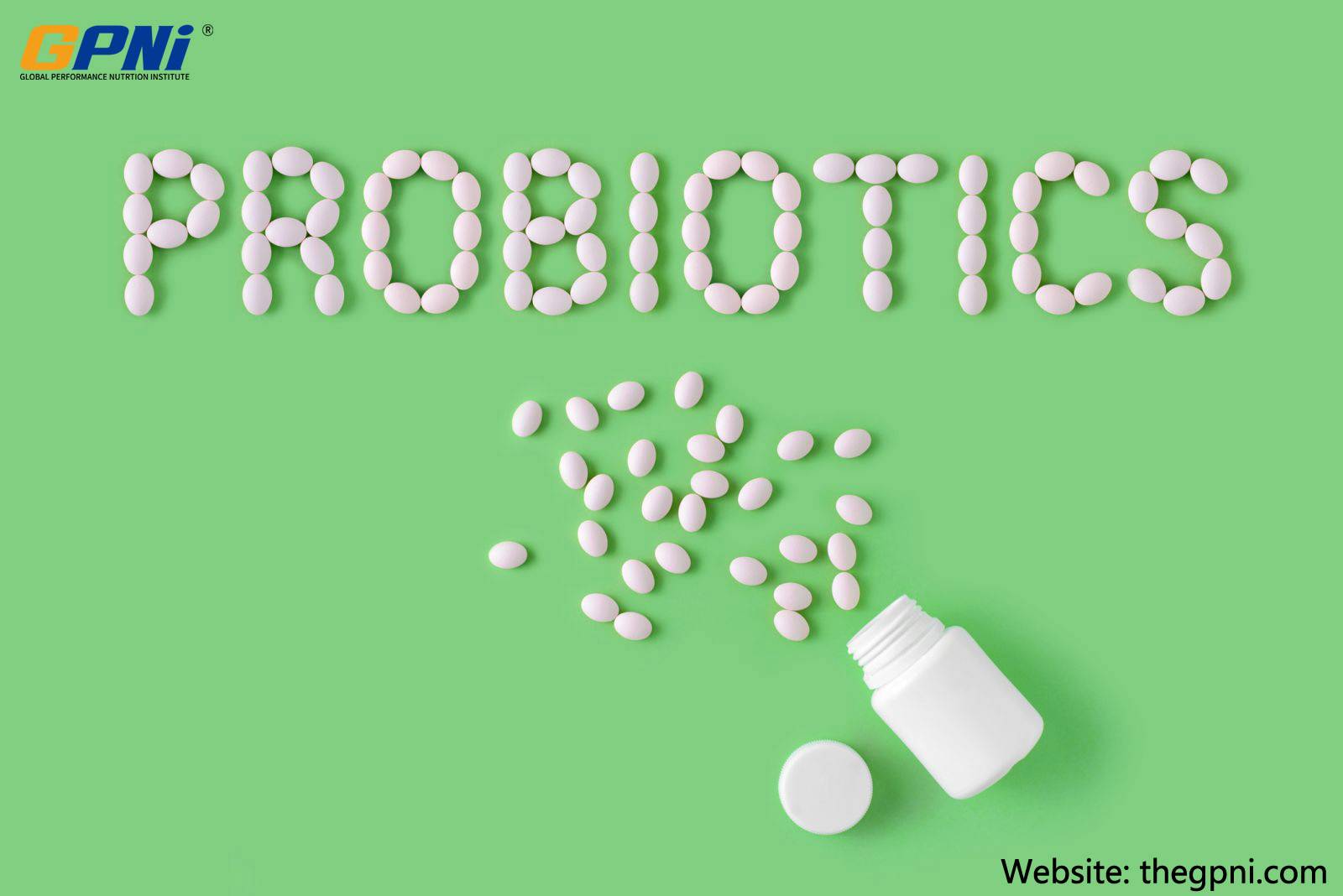
Introduction
The term probiotic is derived from the Latin preposition “pro,” which means “for” and the Greek word “biotic” meaning “life”. Probiotics are widely considered to be health-promoting microorganisms. As outlined in Table 1 and as defined by the World Gastroenterology Organization (WGO), various ingredients can function in probiotic, prebiotic, and symbiotic roles. The Food and Agriculture Organization of the United Nations (FAO) and the World Health Organization (WHO) defines probiotics as “live microorganisms that, when administered in adequate amounts, confer a health benefit on the host” [1]. Additionally, the International Olympic Committee (IOC) has stated that, “Probiotics are live micro-organisms that when administered orally for several weeks can increase the numbers of beneficial bacteria in the gut. These have been associated with a range of potential benefits to gut health, as well as modulation of immune function” [5]. Unique in comparison to other dietary supplements, probiotic preparations contain live, viable, defined microorganisms in sufficient numbers to provide beneficial health effects [6]. Table 1 provides an overview of common definitions and classifications related to probiotic research.
Table 1 Definitions of common terminology and classifications in probiotic research
Concept
Definition
Probiotics
Live microorganisms which, when administered in adequate amounts, confer a health benefit on the host [1].
Prebiotic
A substrate that is selectively utilized by host microorganisms conferring a health benefit on the host [2].
Synbiotics
A synbiotic product beneficially affects the host in improving the survival and implantation of live microbial dietary supplements in the gastrointestinal tract by selectively stimulating the growth and/or activating the metabolism of one or a limited number of health-promoting bacteria [3].
Postbiotics
Postbiotics are bioactive components produced by beneficial bacteria (through a natural fermentation process) which have biological activity in the gut (e.g. short-chain fatty acids) [4].
Immunobiotics
Inactivated probiotics (e.g. heat-killed), in which the dead cells maintain their immune benefit.
Gut
The gastrointestinal tract is a long tube that starts in the mouth and ends at the anus. Its main function is to process food. Approximately 70% of antibody producing cells are is located in the digestive system.
Microbiota vs. Microbiome
The gut microbiota is a diverse ecosystem consisting of bacteria, archaea, viruses, protists and fungal communities (mycobiome) living in the human gut. Microbiome refers to the collection of genomes from all microorganisms in a particular environment
Transient vs. Resident Strain
Supplementary probiotics are transient strains. There is currently no evidence that supplementary probiotics can permanently colonize in the gut as resident strains resist colonization by transient strains. Transient probiotics strains may have numerous beneficial health effects by positively interacting with the immune system or stimulating growth of beneficial resident strains.
Alpha-Diversity
Represents the number of species and the proportion in which each species is represented in the microbiota. A high alpha diversity is present when there is a high number of species and their quantities are alike.
Beta-Diversity
Beta-diversity broadly reflects the species composition diversity between regional and local sites. The beta diversity measures the turnover of species between two regions in terms of gain or loss of species
Classes of probiotics
Definition
Lactic acid bacteria (LAB)
Nonpathogenic, nontoxigenic, Gram-positive, fermentative bacteria that are associated with the production of lactic acid from carbohydrates. LAB grow anaerobically, but unlike other anaerobes, most can grow in the presence of oxygen. Examples include Lactobacillus (ssp. acidophilus, fermentum, plantarum, rhamnosus, casei, reuteri, gasseri), Streptococcus (e.g. salivarius, thermophilus) and Lactococcus.
Bifidobacteria
Bifidobacteria are among the first microbes to colonize the human gastrointestinal tract. Examples include Bifidobacterium bifidum, longum, animalis, and breve. Bifidobacteria are not LAB. They are, however lactic acid producing bacteria (but through a very different metabolic pathway).
Spore-forming bacteria
Soil-based probiotics, also referred to endospores, are the dormant form of bacteria that are highly resistant to physical and chemical influences. Upon ingestion, these spores have a high survival rate through the stomach and germinate in the small intestine. Examples include Bacillus (e.g. coagulans, subtilis). Spore forming bacteria are not necessarily of soil origin. They can also be found in fermented foods.
Yeast
Examples include Saccharomyces boulardii.
The probiotic principle dates back to over 100 years ago. In 1908, Elie Metchnikoff [7] suggested that it would be possible to modify the microbiota in our bodies and replace harmful microbes with useful microbes. Reported health benefits of probiotics include modulation of the immune response, maintenance of the intestinal barrier, antagonism of pathogen adhesion to host tissue, and production of different metabolites such as vitamins, short-chain fatty acids (SCFAs), and molecules that act as neurotransmitters involved in gut–brain axis communication [8]. In the last several decades, research in the area of probiotics has progressed considerably and significant advances have been made in the selection and characterization of specific probiotic cultures. A growing number of dietary supplements containing probiotics are commercially available worldwide, and the number of products being marketed to improve the health and performance of athletes continues to increase substantially. To appropriately describe a probiotic, the genus, species, and strain of each live microorganism (see Table 2) must be detailed on a product label. Additionally, the product label should include the total estimated quantity of each probiotic strain at the end of the product’s shelf life, as measured by colony forming units (CFU) or live cells. Moreover, only a 70% DNA-DNA reassociation is needed for strains to be regarded as the same species [9]. The difference between a Homo sapiens and its most closely related species, the chimpanzee (Pan troglodytes) is 98.4%. Reassociation rates of humans with other primates like Gorilla (97.7%), Orangutan (96.5%), Siamang gibbon (95.5%), and the Hamadras baboon (92.7%) are also relatively high. Further, Lemur (78%) are still within the range for probiotics to be considered the same species (see Fig. 1). Analyzing potential health benefits of probiotics must occur on a strain level, and consumption of probiotic products only disclosing genus and species, but not the strain, on the label should be discouraged.
Table 2 Example illustrating the names of a bacterium (L. rhamnosus GG) at different taxonomic levels
Taxonomic level
Name
Domain
Bacteria
Phylum
Firmicutes
Class
Bacilli
Order
Lactobacillales
Family
Lactobacillaceae
Genus
Lactobacillus
Species
Lactobacillus rhamnosus
Strain
Lactobacillus rhamnosus GG
Fig. 1
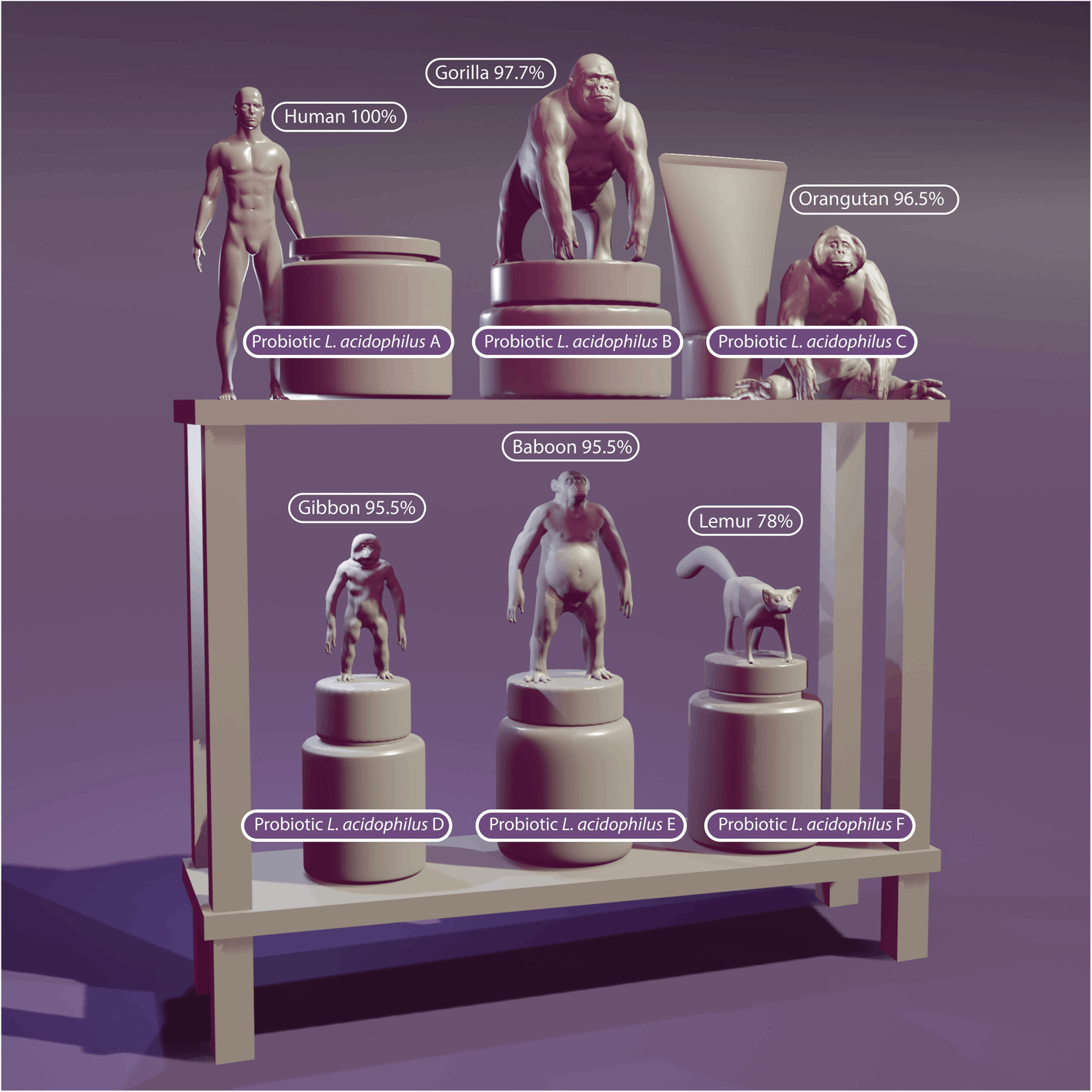
Probiotic benefits are strain specific and probiotics must be described as genus, species and strain, as genetic variation between the same genus and species can be as significant as the difference between a human and a lemur (illustration by Stephen Somers, Milwaukee, WI, USA)
Probiotics are available commercially in capsule or tablet forms, as powder sachets, in the form of liquids and in specific foods such as yogurt and nutrition bars. While fermented foods, such as sauerkraut or kimchi, contain live microbes, they are currently not classified as probiotics, as those products have not been sufficiently studied for their health benefit as stipulated by the definition of probiotics. Stability concerns during manufacture and shelf-life limit food and supplement delivery forms. Probiotics exhibit strain-specific differences in their ability to colonize the gastrointestinal (GI) tract, clinical efficacy, and the type and magnitude of benefits to health in a range of different population cohorts [10]. The effects of probiotics in athletes have been less described in comparison to animal studies and human clinical conditions in the general population. However, the body of probiotic research in recreational and competitive athletes is expanding, including investigations in GI health, exercise performance, recovery, physical fatigue, immunity, and body composition.
Role of diet and exercise on an athlete’s gut microbiome
Numerous factors such as age, genetics, drug use, stress, smoking, and especially diet can all affect the gut microbiome, influencing a complex ecosystem that is highly dynamic and individual [11,12,13,14]. In relation, physical activity has been an area of growing interest in gut microbiome research and appears to promote a health-associated microbiota. In the context of athletes, the present body of literature suggests their microbiota has several key differences in comparison to other populations, likely driven, in part, by exercise and diet. Indeed, several observational studies have investigated the difference in the composition of the gut microbiota between those who are highly physically active (including athletes) and a range of other populations. Reported results include that a higher abundance of health-promoting bacterial species [15,16,17], increased microbiome diversity [16, 18], and greater relative increases in metabolic pathways (e.g. amino acid and antibiotic biosynthesis and carbohydrate metabolism) and fecal metabolites (e.g. microbial produced SCFAs; acetate, propionate, and butyrate) are associated with enhanced fitness [17, 19].
The current evidence supports the role of exercise as an important behavioral factor that can affect qualitative and quantitative changes in the gut microbial composition with benefit to the host. Exercise appears to be able to enrich microbiota diversity [20,21,22,23,24,25], increase the Bacteroidetes-Firmicutes ratio [23], stimulate the proliferation of bacteria which can modulate mucosal immunity [26], improve barrier functions [27], and stimulate bacteria capable of producing substances that protect against GI disorders [28, 29]. Recent research provides further evidence for a role of exercise in shaping the microbiome, with elite runners having a greater abundance of Veillonella that appears to confer a metabolic advantage for endurance exercise by converting exercise-induced lactate to propionate. Pre-clinical studies with Veillonella show a 13% increase in endurance performance [30]. It is likely that the diverse, metabolically favorable intestinal microbiome evident in the elite athlete is the cumulative manifestation of many years of high nutrient intake and high degrees of physical activity and training throughout youth, adolescence and during adult participation in professional sports [31].
In researching the human gut microbiota, it is difficult to examine exercise and diet separately as this relationship is compounded by changes in dietary intakes that often are associated with physical activity (e.g., increased protein intake in resistance trained athletes or carbohydrate intake in endurance athletes and increased total energy and nutrient intake in general). Furthermore, comparing the microbiota of non-athletes to athletes and ascribing any observed differences to exercise alone is not advisable. Athletes generally consume a diet that differs from the general population that has implications for the composition of the gut microbiome.
Diet is an established modulator of gut microbiota composition, with significant change reported within 24 h of a dietary modification [32]. Various food components, dietary patterns, and nutrients all have the potential to alter considerably the growth of different gut microbial populations. Partitioning of individuals into enterotypes appears to be driven by whether their primary dietary patterns include high complex carbohydrate (Prevotella) or high fat/protein (Bacteroides) consumption [33]. Protein intake appears to be a strong modulator of the microbiota [20, 32, 34], with whey protein showing some potential benefits that need further study in humans [31, 35]. Carbohydrates are well known for their profound effect on the gut microbiota, with increased intake of dietary fiber associated with microbial richness and/or diversity [36, 37]. In athletes, higher intakes of carbohydrates and dietary fiber appear to be associated with increased abundance of Prevotella [17, 38]. The specific effects of fat on the gut microbiota is difficult to isolate, however, the types of fats consumed appear to be important [39]. Increased fat intake may promote higher concentrations of bile-tolerant bacteria (presumably because an extremely high fat intake is known to increase bile acid secretion) [32]. Further research is needed to determine the synthesis kinetics and clinical consequence of bile acids and their by-products during increased nutritional intake and metabolic demands during exercise.
Based on the current body of evidence, the athlete gut microbiome may possess a functional capacity that is primed for tissue repair and a greater ability to harness energy from the diet with increased capacity for carbohydrate, cell structure, and nucleotide biosynthesis [19]. This assertion reflects the significant energy demands and tissue adaptation that occurs during intense exercise and elite sport. It appears that being physically active is another important factor in the relationship between the microbiota and host metabolism. Intervention-based studies to delineate this relationship will be important and may provide further insights into optimal therapies to influence the gut microbiota, and its relationship with health and disease as well as athletic performance. Fig. 2 illustrates that an athlete’s gut microbiota is different from a sedentary individual with increased diversity and greater abundance of health promoting bacterial species linked to exercise and increased protein intake.
Key Points 1 – Role of diet and exercise on an athlete’s gut microbiome.
• Active individuals appear to display a higher abundance of health-promoting bacterial species and increased microbiota diversity.
• Body composition and physical activity are positively correlated with several bacterial populations.
• Overall exercise can enrich the microbiota diversity, increase the Bacteroidetes-Firmicutes ratio, stimulate the proliferation of bacteria which can modulate mucosal immunity, and improve barrier functions.
• Diet is an established modulator of gut microbiota composition and activity, with marked changes in microbiota composition evident within 24 h of a dietary modification.
• Protein intake appears to be a strong modulator of microbiota diversity, with whey protein showing some potential benefits that need further study in humans.
• Higher intakes of carbohydrate and dietary fiber in athletes appear to be associated with increased abundance of Prevotella.
• The specific effects of fat on the gut microbiota is difficult to isolate, however, the types of fats consumed appear to be important.
Fig. 2
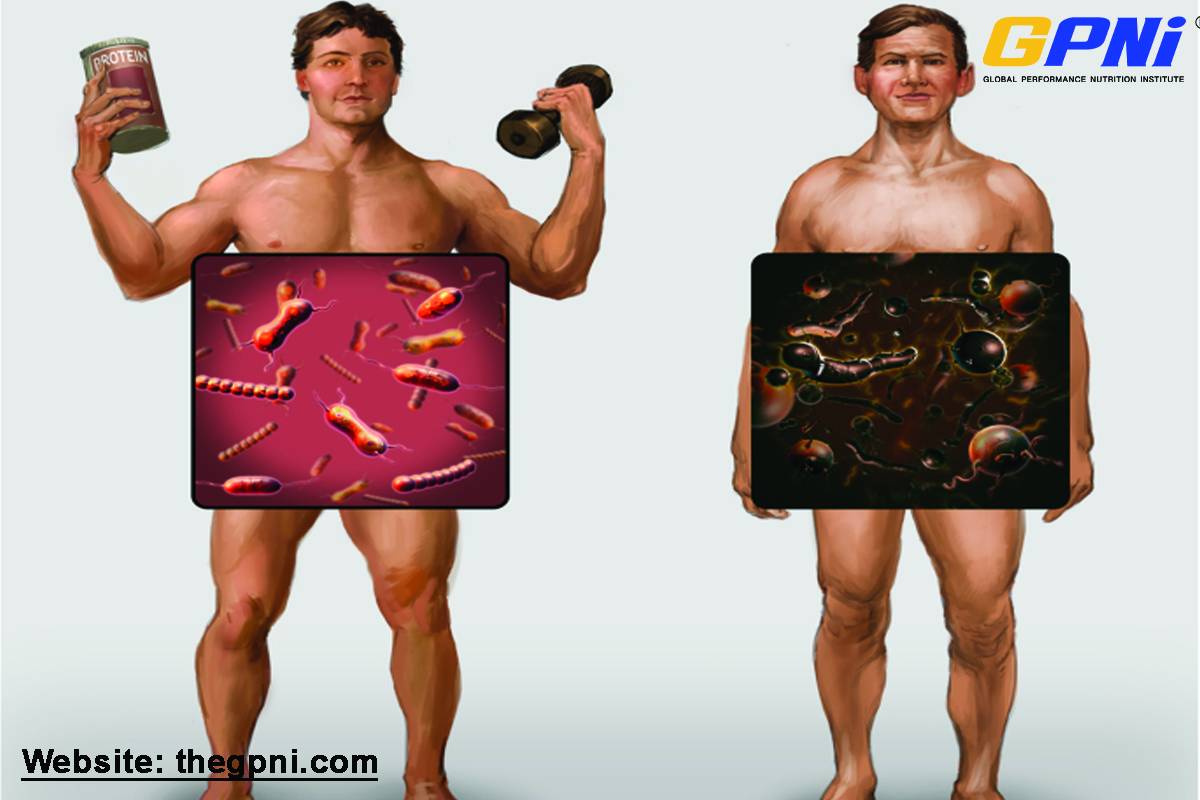
Early research indicates that gut bacteria reflect the activity level of its host. An athlete’s gut microbiota is different from a sedentary individual: increased diversity and greater abundance of health promoting bacterial species linked to exercise and increased protein intake (illustration by Stephen Somers, Milwaukee, WI, USA)
Benefits of probiotic supplementation in athletes
Strenuous and prolonged exercise places stress on the GI tract that increases the likelihood of multiple symptoms associated with a disturbed gut microbiota and decreased performance [40], including abdominal cramping, acid reflux (heartburn), nausea, vomiting, diarrhea, and permeability of the gut that may precipitate systemic endotoxemia [41]. As a major gateway for pathogen entry, the GI tract is heavily protected by the immune system. Modulation of the immune system to increase defenses against upper respiratory tract infection (URTI) is the potential benefit of probiotics for athletes that has been most extensively researched [40]. The microbiome may also have indirect functional influence on various indices of exercise performance and recovery [42,43,44,45,46]. Therefore, probiotics as functional modulators of the microbiome can potentially promote health, exercise adaptation, and performance in athletes.
Probiotics may regulate the mucosal immune response [47], improve the activity of macrophages [48] and modulate the expression of the genes associated with macrophage activity. Probiotics may also interact with Toll-like receptors (TLRs) and downregulate the expression of nuclear factor (NF)-κB and pro-inflammatory cytokines [49, 50]. Additionally, levels of anti-inflammatory cytokines and immunoglobulins, immune cell proliferation, and production of pro-inflammatory cytokines by T cells may be modulated following probiotic supplementation [51, 52]. However, it is often difficult to study athletes during training and competition, and a wide range of interactions between diet, physical activity and other lifestyle stresses needs to be considered. Understanding whether probiotics play a role in athletic performance is of particular interest to athletes who work to improve their results in competition as well as reduce recovery time during training. Moreover, this knowledge may be relevant and of direct benefit to general human health.
The study of probiotic supplementation in athletes and physically active individuals is quite new with the first study in humans published by Clancy et al. [53]. Over the last 13 years, the popularity and number of publications has increased substantially (see Table 3). The number of products containing probiotics directed towards those that exercise is increasing.
Table 3 Probiotic studies in an athletic population: performance, immune and GI health
Reference
Subject group
Sex and age (M ± SD)
Supplementation
Treatment duration
Exercise
Diet
Performance Benefit
Immune or GI Benefit
Clancy et al. (2006) [53]
Healthy recreational athletes (n = 18), Fatigued recreational athletes (n = 9)
11 M / 7 F
16–37 y
6 M / 3 F
17–40 y
L. acidophilus (LAFTI®L10), capsules, 2 × 1010 CFU Daily
4 weeks
Not reported
Not reported
Not assessed
T cell deficit was reversed (increased secretion of IFNƴ from T cells) following probiotic supplementation
Moreira et al. (2007) [54]
Non-elite Marathon runners (n = 141)
62 M / 8 F in treatment group 39 ± 9 y
L. rhamnosus GG (LGG), milk-based drink, 4 × 1010 CFU Daily
12 weeks
Running During pollen season & 2003 Helsinki City Marathon
Subjects instructed to refrain from eating food containing probiotics
Not assessed
No effects on symptoms of atopy or asthma
Non-elite Marathon runners (n = 141)
62 M / 8 F in treatment group 39 ± 9 y
L. rhamnosus GG (LGG), milk-based drink, 4 × 1010 CFU Daily
12 weeks
Running During pollen season & 2003 Helsinki City Marathon
Subjects instructed to refrain from eating food containing probiotics
Not assessed
No effect on respiratory infections or GI episodes. Shortened GI stress post marathon
Tiollier et al. (2007) [56]
French commando cadets (n = 47)
47 M
21 ± 0.4 y
L. casei DN- 1 14 00 1, milk-based drink during training (dose not indicated) Daily
3 weeks
Military training for 3 weeks followed by a 5-day combat course
Military ration. No fermented dairy products
Not assessed
No effect on respiratory tract infections
Cox et al. (2010) [57]
Elite male distance runners (n = 20)
20 M
27.3 ± 6.4 y
1.2 × 1010 CFU L. fermentum VRI-003 (PCC) Daily
16 weeks
Running (winter training)
Not reported
No changes in running performance
Significant reduction in respiratory episodes and severity
Martarelli et al. (2011) [58]
Amateur cyclists (n = 24)
24 M
32.03 ± 6.12 y
L. rhamnosus IMC 501®, L. paracasei IMC 502® 1 × 109 CFU Daily
4 weeks
Intense physical activity
Diets proportionally equivalent in macro and micronutrient quantity, containing 100% of the RDA for all nutrients
Not assessed
Reduced exercise induced oxidative stress
Recreationally active endurance athletes (n = 84)
54 M / 30 F
27.0 ± 11.6 y
L. casei Shirota (LcS), 6.5 × 109 CFU 2x daily
16 weeks
Running (winter training, normal training load)
Consumption of supplements, additional probiotics, or any fermented dairy products were not permitted during the study period
Not assessed
Significant reduction in frequency of URTI
West et al. (2011) [61]
Competitive cyclists (n = 80)
64 M / 35 F
35 ± 9 and 36 ± 9 y
L. fermentum (PCC®) 1 × 109 CFU Daily
11 weeks
Cycling (winter training, normal training load)
Subjects were asked to maintain a normal diet and refrain from eating probiotic or prebiotic enriched foods or supplements
No effect on peak power or VO2 max
Significant reduction in URTI (duration and severity) in males. No effect in females
Välimäki et al. (2012) [62]
Marathon runners Placebo (n = 58), Probiotic (n = 61)
105 M / 14 F
40 (23–69) y
40 (22–58) y
L. rhamnosus GG (LGG), 4 × 1010 CFU Daily
12 weeks
Running training; marathon run
Instructed to refrain from eating food containing probiotics and advised to follow normal dietary habits
Not assessed
No effects on serum LDL or antioxidant levels
Lamprecht et al. (2012) [63]
Endurance trained men (triathletes, runners, cyclists) (n = 23)
23 M
37.6 ± 4.7 y
Multispecies probiotic (B. bifidum W23, B. lactis W51, E. faecium W54, L. acidophilus W22, L. brevis W63, and L. lactis W58, 1 × 1010 CFU Daily
14 weeks
Normal training load
7-dayfood record. Instructed to maintain their habitual diet
No effect on VO2 max, maximum performance
Significant reduction in Zonulin (marker of gut permeability)
Gleeson et al. (2012) [64]
Highly active individuals (n = 66)
28 M / 38 W
23.9 ± 4.7 y
L. salivarious, 2 × 1010 CFU Daily
16 weeks
Endurance-based physical activities (spring training)
Consumption of supplements, additional probiotics, or any fermented dairy products was not permitted
Not assessed
No effect on frequency, severity and duration of upper respiratory tract infections
Grobbelaar et al. (2012) [65]
Moderately active individuals (n = 50)
50 M
18–30 y
Bifidobacterium and Lactobacillus strains (dose not indicated) Daily
6 weeks
Moderately active as defined by ACSM and CDC
Nutritional supplementation prohibited
Not assessed
No significant increases in performance related blood markers
West et al. (2012) [66]
Active individuals (n = 22)
22 M
33.9 ± 6.5 y
Multi-strain probiotic (4.6 × 108 CFU L. paracasei subs paracasei (L. casei 431®), 6 × 108 CFU B. animalis ssp. lactis (BB-12®), 4.6 × 108 CFU L. acidophilus LA-5, 4.6 × 108 CFU L. rhamnosus GG Daily
3 weeks
Recreational cycling
Not reported
Not assessed
No effect on measures of systemic or mucosal immunity including gut permeability
Salarkia et al. (2013) [44]
Adolescent endurance swimmer (n = 46)
46 F
13.8 ± 1.8 y
Multi-strain probiotic yoghurt (L. acidophilus SPP, L. delbrueckii bulgaricus, B. bifidum, and S. salivarus thermnophilus) 4 × 1010 CFU Daily
8 weeks
Swimming
Advised to refrain from other probiotic products
Significant improvement in VO2 max. No effect on swim times
Significant reduction in respiratory and ear infections. No effect on GI episodes
Charlesson et al. (2013)
Abstract of 2012 IJSNEM Confer.
Male athletes (n = 8) (travelling to high risk travelers’ diarrhea countries)
8 M
Age not reported
L. acidophilus, B. lactis, L. rhamnosus (dose not indicated) Daily
8 weeks
Normal training
Not reported
Not assessed
No effect on travelers’ diarrhea (TD). 50% of all athletes reported TD symptoms
Sashihara et al. (2013) [67]
University-student athletes (n = 44)
44 M
Grp-1: 19.8 ± 0.9 y
Grp-2: 19.9 ± 0.9 y
Grp-1: L. gasseri OLL2809 1 × 109 CFU. Grp-2: alpha-lactalbumin 900 mg +: L. gasseri OLL2809 1 × 109 CFU 3x daily
4 weeks
Normal training load
Not reported
No improvement in 1 h of cycle ergometer exercise performance
Prevented reduced natural killer cell activity due to strenuous exercise and elevated mood from a depressed state (POMS)
West et al. (2014) [68]
Active individuals (n = 465)
241 M / 224 F
35 ± 12 y / 36 ± 12 y
B. animalis subsp. lactis BI-04 2 × 1010 CFU, or L. acidophilus NCFM and B. animalis subsp. lactis BI-07 5 × 109 CFU Daily
150 days (21.42 weeks)
Normal activity load (approx. 6 h per week)
Refrain from consumption of non-study probiotic or prebiotic supplements or foods during the study.
Not assessed
BI-04 reduced upper respiratory tract infection frequency. BI-07 + LA NCFM showed no effect. Probiotic treatments delayed URTI ~ 0.8 months
Haywood et al. (2014) [69]
Highly-trained rugby union players (n = 30)
30 M
24.7 ± 3.6 y
L. gasseri 2.6 × 109 CFU, B. bifidum 0.2 × 109, and B. longum 0.2 × 109 CFU Daily
4 weeks
Normal training load (during the winter months)
Asked to maintain a normal diet and refrain from consuming probiotic and prebiotic enriched foods or supplements
Not assessed
Significant reduction in episodes of illness. No effect on illness severity
Shing et al. (2014) [46]
Runners (n = 10)
10 M
27 ± 2 y
Multispecies probiotic (L. acidophilus, L. rhamnosus, L. casei, L. plantarum, L. fermentum, B. lactis, B. breve, B. bifidum, and S. thermophilus) 4.5 × 1010 CFU Daily
4 weeks
Normal training load
Provided with a high glycemic index, low sucrose diet for the 26 h prior to each time to-fatigue run.
Significant increase in run time to fatigue in the heat
No effects on inflammation or GI markers
Aghaee et al. (2014) [70]
Abstract
Athletes (n = 16)
16 M
19–25 y
Probiotic (type and dose not indicated) Daily
30 days
Normal training load
Not reported
Not assessed
Probiotic treatment significantly increased monocyte levels in comparison to placebo control
Georges et al. (2014) PILOT [71]
Resistance-trained individuals (n = 10)
10 M
22.0 ± 2.4 y
B. coagulans GBI-30, 6086 (BC30), 5 × 108 CFU plus 20 g of casein 2x daily
8 weeks
Periodized resistance training (4x per week)
Macronutrients were controlled to 50% carbohydrate, 25% protein, and 25% fat between groups.
Trend to increase vertical jump power (not significant).
Not assessed
Narimani-Rad et al. (2014) [72]
Professional bodybuilding athletes (n = 14)
14 M
20–55 y
Multi-strain probiotic (L. casei 5.1 × 109 CFU/g, L. acidophilus 2 × 109 CFU/g, L. C. 5.1 × 109 CFU/g, L. bulgaricus 2 × 108 CFU/g, B. breve 2 × 1010 CFU/g, B. longum 7 × 107 CFU/g, S. thermophilus 5.1 × 109 CFU/g) Daily
30 days
Normal training load
Not reported
Not assessed
Stimulated thyroid activity. Significant increase in T4 and significant decrease TSH levels. No significant difference in T3 levels
Muhamad & Gleeson (2014) [73]
Active University students (n = 11)
11 (sex not reported) 22 ± 1 y
14 strain probiotic (L. acidophilus, L. delbrueckii ssp. bulgaricus, L. lactis ssp. lactis, L. casei, L. helveticus, L. plantarum, L. rhamnosus, L. salivarius ssp. salivarius, B. breve, B. bifidum, B. infantis, B. longum, B. subtilis, and S. thermophilus.) 6 × 109 CFU Daily
30 days
Not reported
Not reported
No significant change in rating of perceived exertion and HR
No significant change in salivary antimicrobial proteins (a measure of mucosal protection)
Salehzadeh (2015) [45]
Endurance athletes (n = 30)
30 M
21 y
200 ml of probiotic yogurt drink S. thermophilus or L. delbrueckii ssp. bulgaricus 1 × 105 CFU/g Daily
30 days
Intense aerobic training
Not reported
Significant increase in VO2 MAX and aerobic power
Significant decrease in serum CRP, significant increase in HDL
O’Brien et al. (2015) [74]
Male and female runners (n = 67)
Not reported 18–24 y
Kefir beverage (probiotic strain and amount not indicated) 2x week
15 weeks
Marathon training program
Not reported
No effect on 1.5 mile run test times
Attenuated increase in inflammation (serum CRP)
Gill et al. (2016a) [75]
Endurance-trained runners (n = 8)
8 M
26 ± 6 y
L. casei 10 × 1010 CFU Daily
7 days
Running exercise in hot ambient temperature
Refrained from alcohol and caffeine for 72 h and exercise for 24 h before preliminary testing sessions and each experimental trial
No difference in exercise performance on a treadmill test and perception of effort
No improvement in salivary antimicrobial protein (mucosal immune protection) or cortisol status over placebo
Gill et al. (2016b) [76]
Endurance-trained runners (n = 8)
8 M
26 ± 6 y
L. casei 10 × 1010 CFU Daily
7 days
Running exercise in hot ambient temperature
Consumption of other probiotics was prohibited outside the study protocol
Not reported
Did not prevent increases in external heat stress-induced circulatory endotoxin concentration or plasma cytokine profile compared with placebo
Jäger et al. (2016) [42]
Recreationally-trained individuals (n = 29)
29 M
21.5 ± 2.8 y
B. coagulans GBI-30, 6086 (BC30), 1 × 109 CFU plus 20 g of casein protein Daily
2 weeks
Muscle-damaging single leg training bout
Subjects provided a standardized meal prior to exercise bout. Three-day dietary recalls were collected
Significantly increased recovery and decreased soreness. Non-significant trend to increase power
Not assessed
Jäger et al. (2016) [43]
Resistance-trained men (n = 15)
15 M
25 ± 4 y
B. breve BR03 5 × 109 live cells (AFU) & S. thermophilus FP4 5 × 109 live cells (AFU) Daily
3 weeks
Normal training up until 72 h preceding muscle-damaging elbow flexor exercise challenge
Refrain from any nutritional supplements or ergogenic aids
Improved isometric average peak torque production and range-of-motion during acute recovery
Significant decrease in marker of inflammation (IL-6)
Roberts et al. (2016) [77]
Recreational triathletes (n = 30)
25 M / 5 F
35 ± 1 y
Multi-strain pro/prebiotic/antioxidant 30 × 109 CFU per day containing 10 × 109 CFU L. acidophilus CUL-60 (NCIMB 30157), 10 × 109 CFU L. acidophillus CUL-21 (NCIMB 30156), 9.5 × 109 CFU B. bifidum CUL-20 (NCIMB 30172) and 0.5 × 109 CFU B. animalis subsp. lactis CUL-34 (NCIMB 30153)/55.8 mg fructooligosaccharides/ 400 mg alpha-lipoic acid, 600 mg N-acetyl-carnitine Daily
12 weeks
Progressive triathlon training program
Maintained habitual dietary intake. Required not to consume any other nutritional supplement
No significant difference in race times
Significant reduction in endotoxin levels
Strasser et al. (2016) [78]
Trained athletes (n = 29)
13 M / 16 F
26.7 ± 3.5 y
Multi-species probiotic (B. bifidum W23, B. lactis W51, E. faecium W54, L. acidophilus W22, L. brevis W63, and L. lactis W58) 1 × 1010 CFU/g Daily
12 weeks
Winter training
Maintain normal diet and avoid anti-inflammatory drugs, antibiotics, additional probiotics and dietary supplements
Did not benefit athletic performance
Limited exercise-induced drops in tryptophan levels and reduced the incidence of URTI
Michalickova et al. (2016) [79]
Elite athletes (badminton, triathlon, cycling, alpinism, karate, savate, kayak, judo, tennis and swimming) (n = 39)
29 M / 10 F
23.15 ± 2.6 y
L. helveticus Lafti L10, 2 × 1010 CFU Daily
14 weeks
Normal training load (during winter)
Subjects maintained normal diet and were asked to avoid fermented milk products and immunomodulatory supplements
No significant differences in exercise performance
Significant reduction in duration of URTI episodes and decreased symptoms in elite athletes
Gleeson et al. (2016) [80]
College athletes (n = 243)
142M / 101F
20.4 ± 0.2 y
Fermented milk beverage containing L. casei Shirota, 6.5 × 109 CFU 2x daily
20 weeks
Normal training load
Supplements that might influence immune function and additional probiotics or fermented dairy were not permitted
Not assessed
Significant reduction in cytomegalovirus and Epstein Barr virus antibody titres, benefiting immune status
Michalickova et al. (2017)
Elite athletes (badminton, triathlon, bicycling, athletics, karate, kayaking, and judo) (n = 30)
24 M / 6 F
23.6 ± 1.9 y
L. helveticus Lafti L10, 2 × 1010 CFU Daily
14 weeks
Normal training load (winter training)
Subjects maintained normal diet and were asked to avoid fermented milk products and immunomodulatory supplements
Not assessed
Supported humoral and mucosal immunity by preserving total salivary Immunoglobulin A level
Gepner et al. (2017)
Soldiers from elite combat unit (n = 26)
26 M
20.5 ± 0.8 y
B. coagulans GBI-30 (BC30) 1.0 × 109 CFU and HMB 3 g Daily
40 days
Strenuous military training 40 days
No additional dietary supplements nor consumtion any androgens or other performance-enhancing drugs
Not assessed
Combined supplementation attenuated IL-6 and IL-10 response and maintained muscle integrity
Marshall et al. (2017) [81]
Marathon competitors (n = 32)
26 M / 6 F
23–53 y
PRO-grp: Multi-strain capsule; L. acidophilus CUL-60 10 × 109 CFU, and L. acidophillus CUL-21 (NCIMB 30156) 10 × 109 CFU), B. bifidum CUL-20 9.5 × 109 CFU and B. animalis subsp. lactis CUL-34 0.5 × 109 CFU, and 55.8 mg fructooligosaccharides. PGLn-grp: L. acidophilus CUL-60 (NCIMB 30157) 2 × 109 CFU, L. acidophilus CUL-21 (NCIMB 30156) 2 × 109, B. bifidum CUL-20 (NCIMB 30172) 0.5 × 109 CFU, B. animalis subsp. lactis CUL-34 (NCIMB 30153) 0.95 × 109 CFU, L. salivarius CUL61 (NCIMB 30211) 5 × 109 CFU, and each 5-g dose also contained 0.9 g L-glutamine. Daily
12 weeks
Marathon training; Marathon race
Not permitted to consume any other commercial supplementation that conflicted with the study parameters
No difference in marathon time to completion compared to control group
No change in immuno-stimulatory heat shock protein (eHsp72) concentrations
Toohey et al. (2018) [20]
Soccer and volleyball Division I college athletes (n = 23)
23 F
19.6 ± 1.0 y
B. subtilis (DE111) 5 × 109 CFU Daily
10 weeks
Offseason resistance training program
No dietary restrictions were placed on the athletes besides abstaining from other supplement use
No effect on physical performance parameters
Significant reduction in body fat percentage
Brennan et al. (2018) [82]
Abstract of 2018 ACSM Confer.
Endurance athletes (n = 7)
(sex not reported) 31 ± 6.1 y
L. salivarius (UCC118) (dose not indicated) Daily
4 weeks
Not reported
Not reported
Not assessed
Exercise-induced intestinal hyperpermeability was attenuated
Townsend et al. (2018) [83]
Division I Baseball Players (n = 25)
25 M
20.1 ± 1.5 y
B. subtilis (DE111) 1 × 109 CFU DailyB. subtilis (DE111) 1 × 109 CFU Daily
12 weeks
Offseason training
Three-day food logs collected on weeks 1, 9 and 12.
No effect on physical performance or body composition
TNF-α concentrations were significantly lower compared to placebo
Antonio et al. (2018) [84]
Active men and women (n = 20)
6 M/ 14 F
30 ± 8 y
B. breve BR03 5 × 109 CFU and S. thermophilus FP4 5 × 109 CFU Daily
6 weeks
Normal training load (aerobic and/or resistance training)
Subjects were instructed to not alter their diet
No effect on body composition
Not assessed
Huang et al. (2018) [85]
Active men and women (n = 20)
16 M
20–40 y
L. plantarum TWK10 1 × 1011 CFU Daily
6 weeks
Not reported
Normal diet maintained and no consumption of any other nutritional supplements
Improved endurance performance and blood glucose concentration in a maximal treadmill running test
Not assessed
Carbuhn et al. (2018) [86]
Division I collegiate female swimmers (n = 17)
17 F Age not reported
B. longum 35,624, 1 × 109 CFU Daily
6 weeks
Offseason training
Three-day food logs collected at baseline and weeks 3 and 6.
No effect on aerobic/anaerobic swim time trials and force plate vertical jump
No effect on cytokine and gastrointestinal inflammatory markers and salivary IgA levels
Huang et al. (2019) [87]
Healthy adult triathletes (n = 34)
Study 1: 18 M, 20.2 ± 0.7 y Study 2: 16 M, 22.3 ± 1.2 y
L. plantarum PS128 3 × 1010 CFU Daily
Study 1: 4 weeks Study 2: 3 weeks
Sprint triathlon (swimming 750 m, biking 20 km, running 5 km).
Before race: 595 kcal (24 g PRO, 16 g FAT, 90 g CHO). In race: 30–40 g CHO and 500–1000 ml water per hour.
Attenuated post-triathlon performance declines. No effect on body composition.
Reduced post-race inflammatory cytokines, reduced oxidative stress, increased plasma BCAA levels.
Pugh et al. (2019) [88]
Health adult marathon runners (ran marathon race quicker than 5 h within the previous 2 years; n = 24)
20 M / 4 F
34.8 ± 6.9 y
L. acidophilus (CUL60 and CUL21), B. bifidum (CUL20), B. animalis subs p. Lactis (CUL34) > 25 billion CFU daily in total, no information on individual strains
4 weeks (pre-race)
Marathon race
Before race: standardized high CHO, low fiber diet. In race: 60 mL CHO gel with 200 mL (15 min before start, 40 min post and every 20 min for the remainder of the race.
No difference in race times.
GI symptom severity during the final third was significantly lower.
Pumpa et al. (2019) [89]
Elite rugby union athletes (n = 19)
19 M
27.0 ± 3.2 y
L. rhamnosus, L. casei, L. acidophilus, L. plantarum, L. fermentum, B. lactis, B. bifidum, S. thermophilus 120 billion CFU daily in total, no information on individual strains 500 mg S. boulardi (added during stage 3)
17 weeks
27-weeks, divided into three stages: 1) control period (10 weeks); 2) domestic competition (7 weeks); 3) international competition (10 weeks).
A national training camp and 3 domestic games (stage one), 6-weeks of domestic competition (stage two), and 8-weeks of international competition (stage three).
Not assessed
No effect on salivary Immunoglobulin A. Salivary cortisol increased. Increase in salivary alpha-amylase levels during stage 3.
Vaisberg et al. (2019) [90]
Amateur marathon runners with previous history of post-race URTI (n = 42)
42 M
39.5 ± 9.4 y
Fermented milk beverage containing L. casei Shirota, 4 × 1010 CFU Daily
30 days (pre-race)
Marathon race
Unknown
Not assessed
Improved airway and systemic immune and inflammatory responses post-marathon. No significant effect on URTI.
The effect of probiotic supplementation on performance
Research specifically designed to investigate the effect of probiotic supplementation on performance has been less common and overall the results are mixed. Earlier studies that reported performance outcomes generally had primary aims related to immunity and GI health. Of the 24 studies that assessed some metric of athletic performance, 17 reported a null effect, while 7 reported significant improvement. However, more recent research indicates that probiotic supplementation can promote improvements in exercise performance through various pathways in athletes and physically active individuals using discrete strains of probiotics.
Some studies have used single probiotic strain interventions. For example, in a 16-week study investigating the effect of Lactobacillus fermentum VRI-003 on the immunity in 20 elite male distance runners, measures of performance (which included training duration, intensity, and VO2 max) did not change significantly [57]. Similarly, in 80 competitive cyclists, 11 weeks of supplementation with L. fermentum (PCC®) had no effect on peak power or VO2 max [61]. Four weeks of supplementation with Lactobacillus gasseri OLL2809 and alpha-lactalbumin in 44 university-student athletes did not improve cycle ergometer performance [67]. Gill et al. [75] did not find a difference in perception of effort during a treadmill test in eight male endurance-trained runners who supplemented with a high-dose of Lactobacillus casei (10 × 1010 CFU). Finally, in 39 elite athletes from various sports, 14 weeks of Lactobacillus helveticus Lafti L10 supplementation during the winter did not elicit significant differences in exercise performance as measured by VO2 max, treadmill performance time, maximal heart rate and heart rate recovery [79]. The single strain interventions used in these five studies did not produce an aerobic performance benefit.
Null findings were similarly reported in several studies investigating the effects of multi-strain probiotics on aerobic performance. For instance, in endurance-trained men, 14 weeks of a multi-species probiotic had no effect on VO2 max and maximum performance [63]. In a study designed to determine the effects of a 30-day period of supplementation with a 14-strain probiotic at rest, and in response to an acute bout of prolonged cycling exercise for 2 h at 60% VO2max in 11 active, healthy adults there was no significant change in rating of perceived exertion and heart rate [73]. In another study assessing the effects of a multi-strain probiotic (along with 55.8 mg fructooligosaccharides, 400 mg alpha-lipoic acid, 600 mg N-acetyl-carnitine) in 30 recreational athletes over 12 weeks of progressive triathlon training no significant differences were found in race times [77]. Marshall et al. [81] investigated the effects of a multi-strain probiotic for 12 weeks of marathon training in a group of 32 marathon competitors and found no difference in marathon time to completion compared to the control group.
However positive results were reported in thirty endurance athletes supplementing with a yogurt drink, either containing Streptococcus thermophilus or Lactobacillus delbrueckii ssp. bulgaricus or no probiotics over 30 days during intense aerobic training. There was a significant increase in VO2max and aerobic power in the Cooper aerobic test [45]. In thirty-three trained athletes, 12 weeks of winter training supplementation with a multi-species probiotic did not benefit athletic performance; however, the training load (hours per week) was higher in those who supplemented with the probiotic blend vs. the placebo group [78]. One explanation for these findings could be that probiotics may enable better performance capabilities and training adherence when the risk of URTI development is reduced, as individuals with fewer episodes of infections such as common colds are able to train more often and harder. Further, Strasser et al. [78], noted that the multi-species probiotic limited exercise-induced reductions in circulating tryptophan concentration. Higher serum tryptophan levels may enhance the tryptophan transport into the brain and support serotonin metabolism, which can influence an individual’s sensation of fatigue and thus potentially affect training adherence and performance [91]. Interestingly, VO2max was positively correlated with pre-exercise serum tryptophan levels at a moderate magnitude, supporting a role of tryptophan metabolism in training performance.
Huang et al. [85], found increased endurance performance and elevated blood glucose concentration following exercise-to-exhaustion after 6 weeks of high dose (1 × 1011 CFU) Lactobacillus plantarum TWK10 (a plant Lactobacillus strain isolated from Taiwanese pickled vegetables) supplementation in healthy male adults. However, as these were untrained males and no aerobic exercise intervention was reported in this study, these data should be interpreted conservatively in relation to endurance athletes. These results might be explained by an anti-inflammatory effect from L. plantarum TWK10 [92] on skeletal muscle and improvement in energy harvest, possibly related to glycogenesis regulation for exercise demand. Interestingly, L. plantarum KX041 can maintain intestinal permeability and exert antioxidant capacity [93]. Moreover, certain strains of L. plantarum activate cell growth signaling pathways in gut enterocytes which in turn increases protein metabolism in the gut [94]. Further, L. plantarum can rescue the shunted growth phenotype in malnourished mice by activating muscle, bone, and organ growth [95].
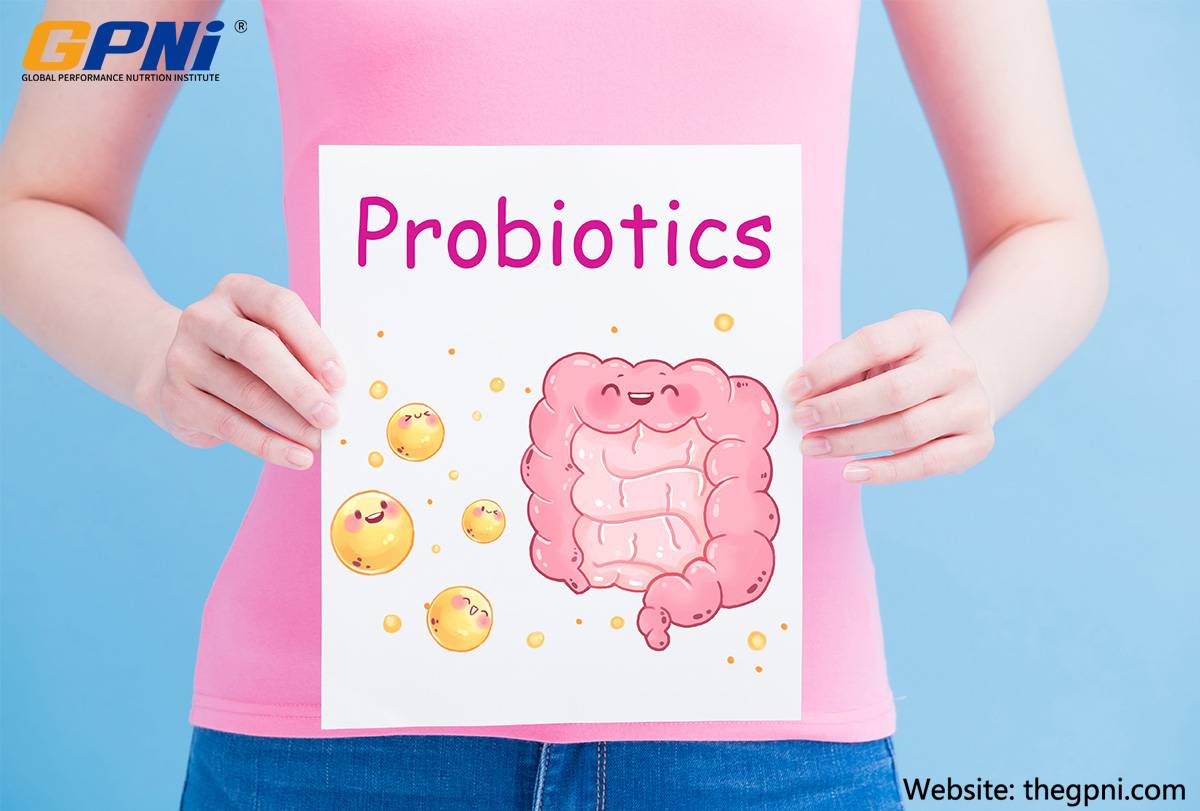
In a study investigating the effect of a multi-strain probiotic yogurt on performance in adolescent female endurance swimmers over 8 weeks, there was a significant improvement in VO2 max [44]. The improvement in VO2 max was attributed to the reduction in number and duration of URTI for athletes following intake of the multi-strain probiotic yogurt. In another study researching the effect of multi-strain probiotics Shing et al. [46] found 4 weeks of supplementation improved time to fatigue while running in the heat for ten male runners. While the mechanism for improvement was unclear, it was speculated that probiotics may exert small to large effects on GI structural integrity, endotoxin translocation and immune modulation that combine to enhance exercise performance. In contrast, a Kefir beverage (a naturally fermented milk beverage containing a defined mixed microbial culture of lactic acid bacteria and yeasts) consumed over 15 weeks of marathon training by sixty-seven male and female runners had no effect on 1.5 mile run test performance [74]. Currently, there are more studies showing a benefit for multi-strain probiotics in relation to performance measures compared to single-strain probiotics. While there are some encouraging results, a large majority of studies have found no effect on aerobic performance. It appears that some of the positive benefits of probiotic supplementation may be indirect by allowing for improved gut integrity or immune modulation. However, additional research is warranted including investigating potential performance outcomes beyond aerobic-based endurance exercise.
Other studies have explored the effect of probiotic supplementation in relation to resistance training on muscle recovery and body composition. A pilot study in ten subjects using resistance trained males supplemented 20 g of casein protein with or without Bacillus coagulans GBI-30, 6086 (BC30) for 8 weeks following a periodized resistance training program showed a trend to increase vertical jump power [71]. Jäger et al. [43] speculated that the potential improvement in vertical jump performance may have been related to improved muscle recovery through gut microbial modulation. In a follow up study, 20 g of casein protein co-administered with B. coagulans GBI-30, 6086 (BC30) or a placebo in recreationally-trained individuals for 2 weeks increased recovery and decreased soreness after a muscle-damaging single-leg training bout [43]. Furthermore, exercise-induced muscle damage was decreased as measured by serum creatine kinase, which may also indicate improved cellular integrity rather than damage per se. While not fully understood, candidate mechanisms of action included the production of digestive enzymes that are active under gut conditions (e.g. alkaline proteases) and these proteases can digest proteins more efficiently than the endogenous human proteases alone [43, 96, 97]. Further, B. coagulans GBI-30, 6086 enhances the health of the cells of the gut lining through improved nutrient absorption including minerals, peptides, and amino acids by decreasing inflammation and encouraging optimum development of the absorptive area of the villi [98]. In vitro, B. coagulans GBI-30, 6086 can increase protein absorption [99]. The combination of B. coagulans GBI-30, 6086 with casein protein may have acted synergistically to augment digestion and modulate absorption.
In fifteen resistance-trained men, 3 weeks of Bifidobacterium breve BR03 and S. thermophilus FP4 supplementation improved isometric mean peak torque production and range-of-motion during acute recovery after a muscle-damaging elbow flexor exercise challenge in comparison to a control group [42]. While mechanisms behind these observations were not described, these strains can have anti-inflammatory effects [100,101,102] and colonize in different areas of the GI tract. However, using the same strains and dose, Antonio et al. [84], failed to see a significant effect on body composition in highly-trained men and women over a longer, six-week period. In both of the above studies participants were not provided supplemental protein. Toohey et al. [103] investigated the effects of Bacillus subtilis DE111 probiotic supplementation on muscle thickness and strength, body composition, and athletic performance in Division I female volleyball and soccer athletes for 10 weeks of an offseason resistance training program. Both groups consumed a protein and carbohydrate recovery drink (consisting of 45 g carbohydrates, 20 g protein, and 2 g fat) immediately after each training session. Probiotic supplementation with the post-workout recovery drink yielded greater reductions in body fat and increases in fat free mass after 10 weeks of resistance training than a placebo. Although no performance advantages were observed, Toohey et al. [103], speculated that supplementation may have promoted improved dietary protein absorption and utilization, contributing to improvements in body composition by increasing dietary protein-induced thermogenesis and altering satiety signaling. It seems that several strains of lactic acid bacteria, including L. gasseri SBT 2055, Lactobacillus rhamnosus ATCC 53103, and the combination of L. rhamnosus ATCC 53102 and Bifidobacterium lactis Bb12, are effective at reducing fat mass in obese humans [104]. Additionally, other strains of B. breve have shown anti-obesity effects in both humans [105] and mice [106].
Townsend et al. [83], evaluated the effect daily B. subtilis (DE111) supplementation on physical and performance adaptations in Division I collegiate baseball players following 12 weeks of offseason resistance training. On training days, placebo or probiotic capsules were consumed immediately post-workout with a protein and carbohydrate recovery drink (consisting of 36 g carbohydrates, 27 g protein, and 2 g fat). There were no group differences observed between those who took the probiotic and placebo for any measure of strength, performance, or body composition. However, those athletes who did supplement with probiotics had significantly lower serum TNF-α concentrations than the placebo group. Elevations in TNF-α have been linked to suppressed protein synthesis, disordered sleep, and impaired muscular performance [107,108,109]. The null performance findings reported by Townsend et al. [83] and Antonio et al. [84] may have been the result of an inability for the probiotic supplement to modify healthy participants’ microbiomes. Indeed, the subjects in these two studies were young, healthy and highly active. In this regard, systematic reviews [110, 111] and an original investigation involving supplementation [112] of probiotic supplementation in adults indicate that probiotic supplementation is more likely to alter the microbiome composition of dysregulated microbiomes compared to healthy ones. While probiotic consumption may not alter microbiome composition, it can alter functionality by up regulation of gene expression and metabolic pathways. As noted for aerobic performance, it is also plausible that probiotic supplementation confers an indirect effect on performance and that the training, diet, and recovery of the individuals in some of these studies were optimal enough to mask any small additional benefits.
Key Points 2 – Probiotic Supplementation and Performance
• To date single-strain probiotic supplementation has produced a significant aerobic performance benefit in only one study.
• Supplementation with multi-strain probiotics has been reported to increase VO2 max, aerobic power, training load, and time to exhaustion in several studies, but more studies have not found such an effect.
• In response to muscle-damaging resistance exercise, probiotic supplementation (paired with protein) can expedite recovery and decrease soreness and other indices of skeletal muscle damage.
The effect of probiotic supplementation on body composition has been mixed and requires further research.
• Probiotics supplementation as an ergogenic aid for performance enhancement requires further investigation and may be indirect via modulation of other systems.
The effect of probiotic supplementation on the immune system
The mucosal lining of the GI tract represents the first-line-of-defense against invading pathogens and is an important interface with the host immune system. Exhaustive physical exercise negatively impacts immunity, reducing of the count and function of immune cells, such as natural killer (NK) cells and T lymphocytes. Pro-inflammatory cytokines such as IL-1, TNF-α and IFN-γ generally remain unchanged after prolonged exercise whereas the inflammation-responsive cytokine IL-6 and anti-inflammatory cytokines such as IL-10, IL-1ra, sTNFR increase markedly. The increase in IL-6 is not solely in response to inflammation in this situation as it also originates from contracting muscle and is associated with glycogen regulation. Gene expression in white blood cells is upregulated for most anti-inflammatory markers and downregulated for pro-inflammatory markers and TLR signaling. The anti-inflammatory hormone cortisol is also elevated [53, 57, 59, 113, 114]. Changes in immune health are associated with increased incidence of URTIs and disorders of the GI tract [46, 53] which have the potential to impair physical performance and/or cause an athlete to miss training or competition [115]. These conditions usually occur during competitive periods that are commonly represented by higher intensities and greater volumes of exercise [116], affecting the athlete’s health and impairing physical performance when needed most [115]. In this context, interventions that prevent or mitigate these conditions can indirectly improve physical and competition performance. Among the nutritional supplements used in modulation of the immune response of athletes, probiotics are noteworthy [92].
Probiotics appear to augment intestinal communication between the host immune system and commensal bacteria to establish mutualistic benefits. The roles of microbial-derived SCFAs, particularly butyric acid in the colon, are important in mucosal homeostasis through regulation of epithelial turnover and induction of regulatory T (Treg) cells [117]. Beyond the GI tract, probiotics have an immunomodulatory effect through the common mucosal immune system, in which cells from inductive sites (e.g., Peyer’s Patches in the intestines) translocate to mucosal surfaces following interaction with antigen-presenting cells [118].
Research investigating the effects of probiotics on immune outcomes have been the most prevalent type of research in athletic populations. Of the 22 studies reviewed in this Position Stand that assessed the effect of probiotics on outcomes related to the immune system, 14 reported significant improvement, whereas 8 reported no effects.
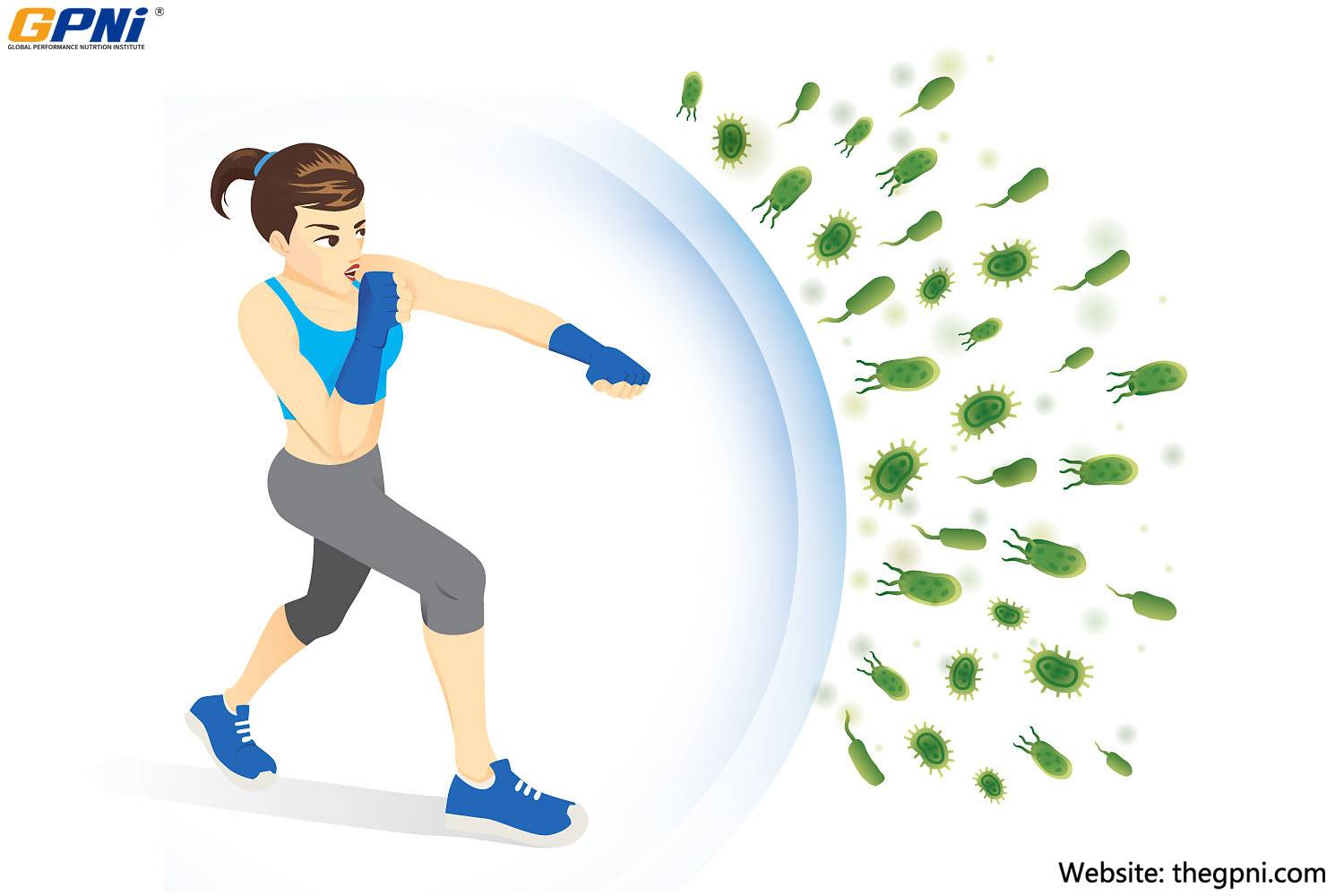
Of particular relevance to athletes is the reduction in incidence and/or severity of symptoms from illnesses like URTI. In a large study of 465 active individuals who had a normal activity load of approximately 6 h per week, West et al. [68] compared a single strain treatment consisting of Bifidobacterium animalis ssp. lactis Bl-04 and double-strain probiotic consisting of Lactobacillus acidophilus NCFM and B. animalis subsp. lactis Bi-07 to placebo over a 150-day intervention. Daily B. animalis ssp. lactis Bl-04 supplementation for 150 days was associated with a 27% reduction in the risk of any URTI episode compared to placebo supplementation. Supplementation with the double-strain probiotic resulted in a 19% decrease of URTI risk, although this was not statistically significant. Moreover, both probiotic supplement groups exhibited a ~ 0.8-month delay in time to illness. Importantly, healthy active individuals with a lighter training load, and presumably at a lower risk for URTIs, also appeared to benefit from a probiotic supplement.
The majority of studies that have investigated the potential benefits of probiotics on URTIs have been conducted in endurance athletes with generally high training loads. For example, Cox et al. [57] studied the effect of L. fermentum VRI-003 (PCC) over 16 weeks of winter training in 20 elite male distance runners on incidence of illness and infection. Probiotic supplementation significantly reduced URTI incidence and severity compared to placebo. Specifically, those in the treatment group reported less than half the number of days of respiratory illness symptoms compared to the control group during the intervention. While not significant, there was a trend for enhanced T-lymphocyte function, which may be in part responsible for the immunological benefits. Similarly, Gleeson et al. [60] examined the effects of Lactobacillus casei Shirota during 4 months of winter training in endurance-based recreational athletes and observed a significant reduction in URTIs compared to placebo. In addition, salivary IgA concentration was significantly higher in those consuming the probiotic. However, severity and duration of symptoms were similar between the treatment and placebo groups. Supplementation with the same strain 30 days prior to a marathon race resulted in improved systemic and airways immune responses, and showed a trend toward improved incidents and duration of URTI post-marathon [90]. In competitive cyclists, West et al. [61] reported reduced severity of self-reported symptoms of lower respiratory illness and use of cold and flu medication over an 11-week winter training period with L. fermentum (PCC®) compared to placebo. Interestingly, this effect was only noted in males and not females. Strasser et al. [78] examined the effect of 12 weeks of treatment with a multi-strain probiotic on the incidence of URTIs and metabolism of aromatic amino acids after exhaustive aerobic exercise in highly trained athletes during the winter. Daily supplementation with probiotics reduced the incidence of URTI compared to placebo. In addition, supplementation limited exercise-induced reductions in tryptophan levels, which may reduce the risk of developing an infection.
Beyond studies investigating traditional endurance athletes with high aerobic training loads, probiotic supplementation has also been examined in other athletes with varying demands. For instance, Salarkia et al. [44] reported that 8 weeks of supplementation with a multi-strain probiotic yogurt reduced the number of episodes of URTIs in adolescent female swimmers compared to the same yogurt without probiotics. Haywood et al. [69] investigated the effect of a multi-strain probiotic over 4 weeks in 30 elite union rugby players to determine effectiveness on the number, duration and severity of infections. The probiotic group had lower incidence of infection-related symptoms compared to placebo, although there was no difference in the severity of the symptoms between the two treatment groups. In a study of an eclectic group of elite athletes training in badminton, triathlon, cycling, alpinism, athletics, karate, savate, kayak, judo, tennis, and swimming, Michalickova et al. [79] studied the effects of L. helveticus Lafti L10 over 14 weeks during the winter. Athletes all had high training loads of > 11 h per week and were winners of the national or European and world championships in their categories and sport. Supplementation with the probiotic significantly reduced the length of URTI episodes and lowered the number of symptoms per episode compared to placebo. Moreover, there was a significant increase of CD4+/CD8+ (T helper/T suppressor) cells ratio in the probiotic group. Previously, this ratio has been noted as an index sensitive to high training loads and was decreased after strenuous physical activity [36, 119]. In addition, low CD4+/CD8+ cell ratio is usually related to acute viral diseases [120].
Several studies that assessed similar outcomes did not report significant effects from probiotic supplementation compared to placebo. For example, a 12-week study on 141 non-elite marathon runners during pollen season supplementing daily with L. rhammnosus GG (LGG) did not find a significant effect on allergic markers [54] or on the incidence of UTRI episodes [55]. Similarly, there was no significant effect on URTI incidence in a study investigating the effect of L. casei supplementation in French soldiers participating in intense military training for 3 weeks in a 5-day combat course [56]. In addition, there was no difference in salivary IgA or total and differential leukocyte and lymphocyte subsets.
Gleeson et al. [64] examined the effects of daily supplementation of L. salivarius on 66 endurance-based recreational athletes during a four-month period in the spring. There was little effect on frequency, severity or duration of URTIs. In addition, circulating and salivary immune markers did not change over the course of the study and were not different between probiotic and placebo groups. Gleeson et al. [80] also assessed the effect of L. casei Shirota on the incidence of URTIs over a 20-week period during the winter in 243 college endurance athletes. Similarly, there was no significant difference between those that consumed the probiotic and the placebo treatment. However, there was a reduction in plasma cytomegalovirus and Epstein Barr virus antibody titers in seropositive athletes compared to placebo, an effect interpreted as a benefit to overall immune status.
While these null findings are important to consider, the current overall body of evidence is weighted notably in favor of probiotics on reduction of URTIs and related symptoms. However, a central issue in relation to the effects of probiotics on immunity, and probiotic research in general, is the large assortment of strains used. Shared, core mechanisms for probiotic function are evident, although some mechanisms may be more narrowly distributed, including those related to immunomodulation [121]. In addition, it is important to note that immune response is complex, as are many of the methodologies used to measure it. For example, an immunomodulatory effect of probiotics is attributed to the release of a large number of cytokines and chemokines from immune cells, which can further impact the innate and adaptive immune systems [122]. Therefore, it is not surprising that the beneficial effect of probiotic administration on the incidence of respiratory illness is possibly linked enhancement of systemic and mucosal immunity. It is possible changes occurred at this level and were not detected in studies that only measured URTI associated metrics. Future work in this area should pair the investigation of URTI incidence and symptomology with other markers of immune response to provide a more thorough understanding of how different probiotics might influence the immune system.
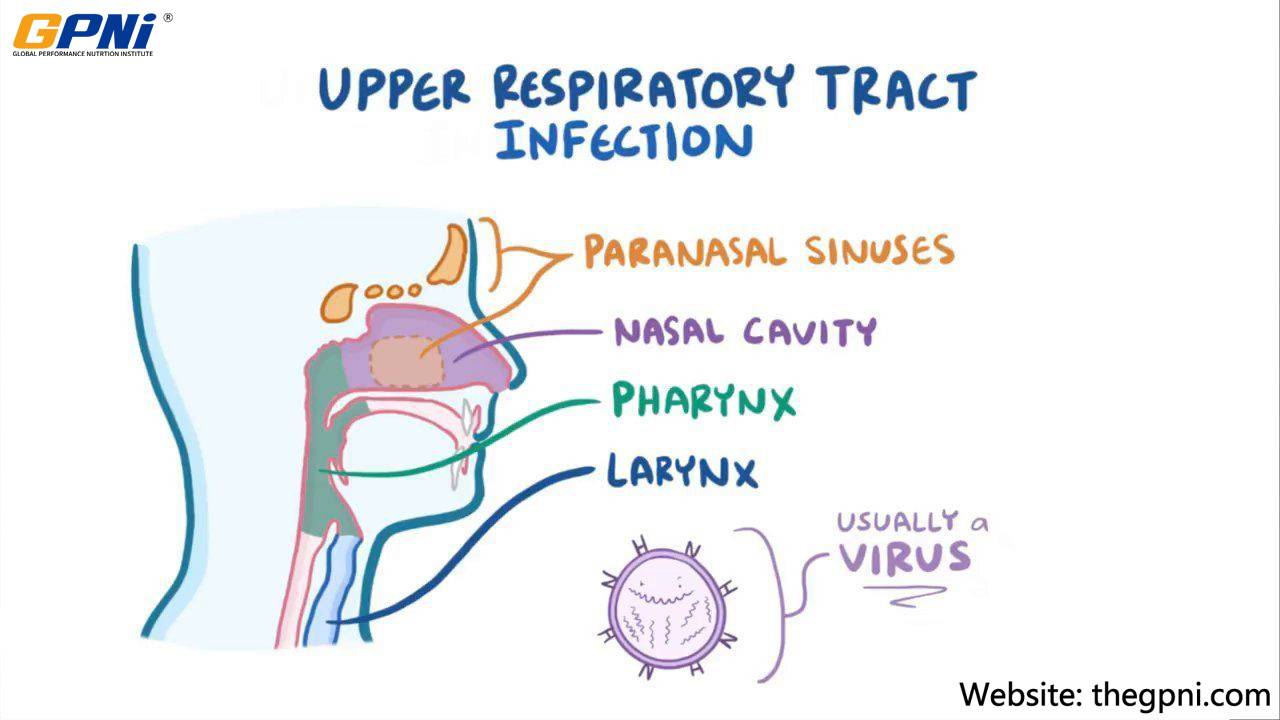
Although less common than symptom outcomes, several studies have provided encouraging evidence in regard to changes in circulating and salivary immune markers. For instance, Clancy et al. [53] sought to determine if immune variables differed between healthy and fatigued recreational athletes after Lactobacillus intervention. One month of daily L. acidophilus supplementation significantly increased secretion of interferon (IFN)-γ from T cells in fatigued athletes to levels found in healthy athletes and increased the concentration of IFN-γ in saliva of healthy control athletes. IFN-γ is a cytokine intricately linked to mechanisms of control of both virus shedding and disease re-activation. Sashihara et al. [67] evaluated the immunopotentiation and fatigue-alleviation effects of L. gasseri OLL2809 supplementation for 4-weeks in 44 university-student athletes. Before and after the treatment period, the subjects performed strenuous cycle ergometer exercise for 1 h. The probiotic supplementation prevented reduced NK cell activity after strenuous exercise which may enhance resistance against infections. In another short-term study, Aghaee et al. [70] reported that a probiotic supplement for 30 days in 16 male athletes increased blood monocyte levels following exhaustive exercise in comparison to placebo control. In a longer duration study, Michalickova et al. [79] investigated the effects of L. helveticus Lafti L10 supplementation on systemic humoral and mucosal immune response in 30 elite athletes with a high training load (> 11 h per week) over 14 weeks in the winter. Those that consumed the probiotic exhibited attenuated decreases in total salivary IgA level compared to athletes in the placebo group. Given the fact that mucosal surface is the first-line-of-defense against different pathogens, this finding might have a practical application in terms of prevention of URTIs during strenuous exercise in elite athletes. In comparison to some of the previous studies that didn’t report changes in immune parameters, yet noted a difference in URTI incidence, it is possible that in these circumstances these strains could have displayed antagonistic activities against pathogens and not direct stimulation of the immune system. These effects could include the production of antimicrobials, such as bacteriocins, and low molecular weight compounds such as hydrogen peroxide, lactic acid, and acetic acid [123,124,125]. These substances could function to outcompete pathogenic bacteria and help in easing or preventing URTI symptoms [126].
In contrast, West et al. [66] did not find significant effects of a synbiotic product including multi-strain probiotics (Lactobacillus paracasei ssp. paracasei (L. casei 431®), B. animalis ssp. lactis (BB-12®), L. acidophilus LA-5, L. rhamnosus GG) on markers of circulating and mucosal immunity in 22 recreational cyclists over a three-week training period. In another small study of the effects of a multi-strain probiotic (L. acidophilus, L. delbrueckii ssp. bulgaricus, Lactococcus lactis ssp. lactis, L. casei, L. helveticus, L. plantarum, L. rhamnosus, L. salivarius ssp. salivarius, B. breve, Bifidobacterium bifidum, B. infantis, Bifidobacterium longum, B. subtilis, and S. thermophilus) on mucosal immunity, Muhamad & Gleeson [73] did not report a significant alteration in salivary antimicrobial proteins at rest or in response to an acute bout of prolonged exercise in 11 active, healthy adults after 30 days of supplementation. Using a high-dose probiotic treatment, Gill et al. [75] studied 8 male endurance runners who consumed 10 × 1010 CFU of L. casei for 7 days prior to a two-hour running exercise at 60% VO2max in hot ambient conditions (34.0 °C and 32% relative humidity). Supplementation did not enhance salivary antimicrobial proteins responses and subsequent oral-respiratory mucosal immune status above placebo. Finally, Carbuhn et al. [86] explored the effects of B. longum 35,624 supplementation in 20 female Division I collegiate swimmers during a 6-week intense training phase on IgA. There were no difference in salivary IgA between groups throughout the study in agreement with a study investigating B. subtilis DE111 in collegiate baseball players [83].
Overall, the effect of probiotic supplementation on the immune system in athletes is likely positive and beneficial. Episodes of illness often occur during heavy exercise training periods, a time when athletes obtain the greatest improvements in fitness. Illness that interrupts individual training sessions may prevent athletes from maximizing the effects of their training program. Therefore, probiotic supplementation may be viewed as a viable dietary supplement to support immune function during these periods.
Key Points 3 – Effects of Probiotic Supplementation on Immune Function
• Athletes may compromise their immune status with high training loads (over-reaching, over-training) which can increase the risk of illness such as URTIs.
• Overall, the current body of evidence indicates small variable benefits of probiotics during intense training, particularly in endurance athletes, the cohort where the majority of studies are conducted.
• There is more evidence for the clinical effects of probiotics reducing the incidence URTI and related illness.
• Positive changes in circulating and salivary immune markers have been more variable and require further research to define more clearly.
The effect of probiotic supplementation on GI tract health
GI problems often occur in endurance athletes and particularly during prolonged events such as cycling, triathlons and marathons [41, 127]. Symptoms such as nausea, cramping, bloating, and diarrhea most likely reflect redistribution of blood flow from the gut to the skin for cooling purposes. Exercise-induced redistribution of blood can result in splanchnic hypoperfusion as a possible mechanism for gut dysfunction [128, 129]. The physical up-and down movement of the gut during running could also explain an increase in the frequency of gut symptoms [41]. Interactions between prolonged exercise, challenging environmental conditions (temperature, altitude, humidity, etc.), and nutrient and fluid intake may also increase risk of gut problems [130]. Disruption in the GI system can impair the delivery of nutrients, and cause GI symptoms and decreased performance. The GI tract and particularly the gut are quite adaptable and can be targeted to improve the delivery of nutrients during exercise while at the same time alleviating some (or all) of the symptoms [131]. A major limitation of studies in this field is that the prevalence of GI illnesses overall is quite low, which makes it difficult to study without a large number of subjects. Probiotic supplementation in combination with other dietary strategies (e.g. consuming well-tolerated foods and drinks, avoiding spicy foods) could assist athletes with a history of GI problems. Moreover, probiotic supplementation potentially could improve GI health which has several indirect athletic benefits. Of the ten studies that assessed GI benefit in athletes and physically active individuals, the majority reported no effect. However, the methodology varied considerably, including probiotic type (species/strain), dosing, duration and study participants, making comparison difficult. Further, the overall result is not conclusive as four studies reported positive results. This latter group included significantly decreased concentrations of zonulin [63] and endotoxin [77], as well as intestinal hyperpermeability [132] and duration of GI-symptom episode. Research in this area has only been conducted intermittently over the past 10 years, with the need for future studies apparent.
Investigating markers of gut permeability, West et al. [66] found no significant effect of multi-strain probiotic supplementation on the lactulose/mannitol ratio in active individuals after 3 weeks. Lamprecht et al. [63] explored the effects of 14 weeks of multi-species probiotic supplementation on zonulin from feces in trained men. Zonulin concentrations decreased significantly from slightly above normal into the physiological range in subjects that supplemented with the probiotics. Zonulin is a protein of the haptoglobin family released from liver and intestinal epithelial cells and has been described as the main physiological modulator of intercellular tight junctions [133]. Increased zonulin concentrations are related to changes in tight junction competency and increased GI permeability [133]. The “leak” in the paracellular absorption route enables antigens to pass from the intestinal environment, challenging the immune system to produce an immune response and subsequent inflammation and oxidative stress [134,135,136]. Lamprecht et al. [63] suggested that the supplemented probiotics may activate the TLR2 signaling pathway resulting in improved intestinal barrier function, thus reducing an athlete’s susceptibility to endotoxemia and associated cytokine production [137].
In the first reported study investigating the effects of probiotics on GI health, Kekkonen et al. [55], reported no effect of L. rhamnosus GG on GI-symptom episodes in marathon runners after a three-month training period. However, the duration of a GI symptom episode was 57% shorter in the probiotic group than in the placebo group. Eight weeks of supplementation with a multi-strain probiotic yogurt in adolescent female endurance swimmers did not affect GI symptoms [44]. In a study of elite union rugby players, subjects given a multi-strain probiotic over 4 weeks did not experience a significant reduction in GI episodes (including nausea, vomiting, diarrhea) compared to the placebo [69].
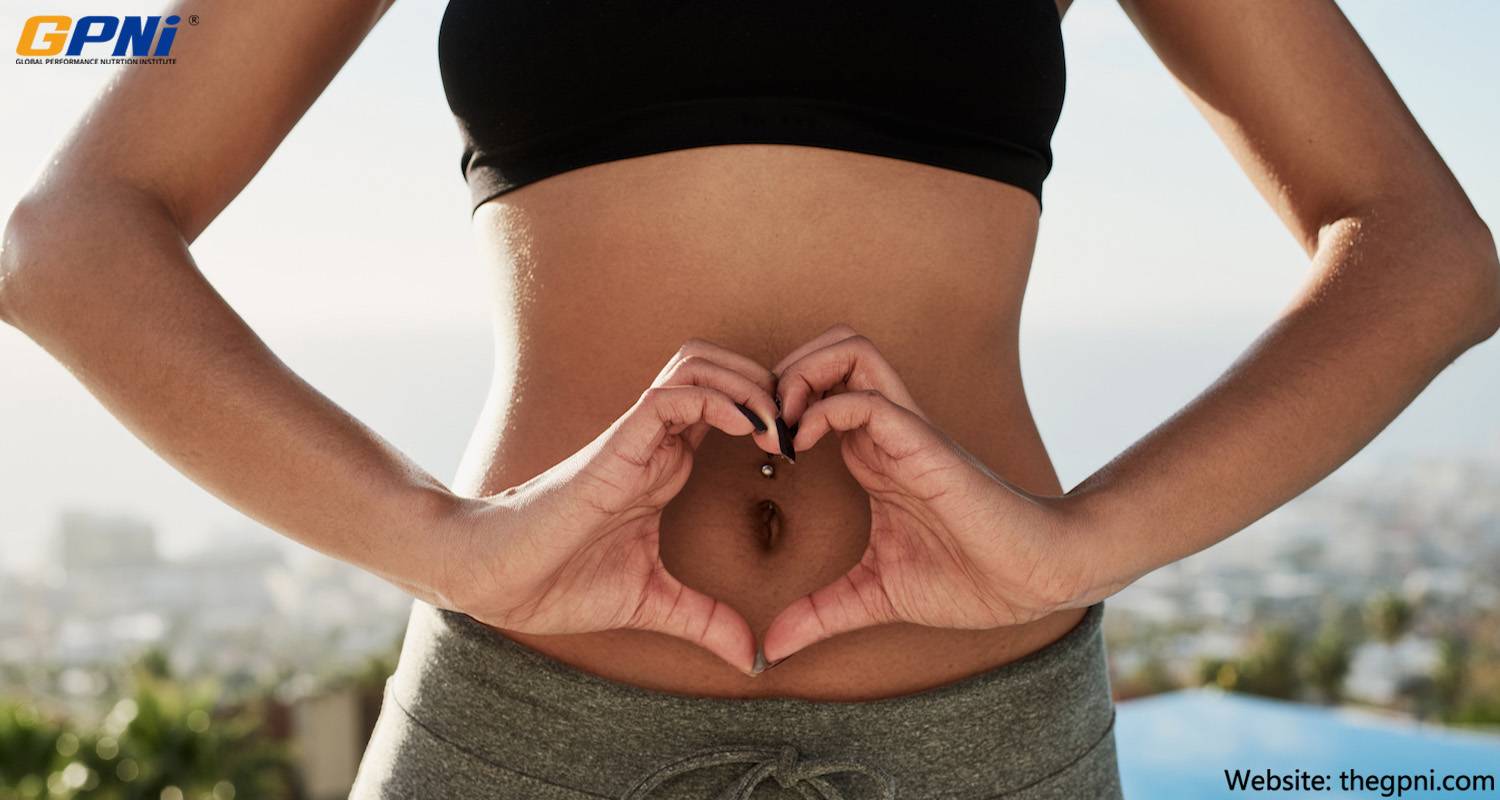
Shing et al. [46] tested the effects of 4 weeks of multi-strain probiotics supplementation on GI permeability when exercising in the heat in a small group of male runners. To assess GI permeability, subjects ingested lactulose and rhamnose before exercise and post-exercise urine was collected to measure the ratio. Further, urinary claudin-3, a surrogate marker of gut barrier disruption, and serum lipopolysaccharide (LPS) were measured. There was no significant effect on lactulose:rhamnose ratio, urinary claudin-3 or serum LPS and it is possible that 4 weeks may not have been sufficient to detect changes. In short-term, high dose single-strain probiotic supplementation (L. casei), male runners under heat stress did not exhibit any marked changes in resting circulatory endotoxin concentration or plasma cytokine profile compared with placebo [76]. Conversely, Roberts et al. [77] reported 12 weeks of supplementation with a multi-strain probiotic/prebiotic significantly reduced endotoxin levels in novice distance triathletes. However, no difference was identified in the assessment of intestinal permeability from urinary lactulose:mannitol ratio. This effect was reported both pre-race and 6 days post-race. Additionally, seven highly-trained endurance athletes who received 4 weeks of L. salivarius (UCC118) attenuated exercise-induced intestinal hyperpermeability [132]. Most recently, 12 weeks of probiotic supplementation (B. subtilis DE111) had no effect on gut permeability as measured by zonulin in Division I baseball players [83].
Key Points 4 – Probiotic Supplementation and Gastrointestinal Health.
• GI problems often occur in endurance athletes and can impair the delivery of nutrients, cause GI symptoms and decrease performance
Investigating markers of gut permeability, West et al. [66] found no significant effect of multi-strain probiotic supplementation on the lactulose/mannitol ratio in active individuals after 3 weeks. Lamprecht et al. [63] explored the effects of 14 weeks of multi-species probiotic supplementation on zonulin from feces in trained men. Zonulin concentrations decreased significantly from slightly above normal into the physiological range in subjects that supplemented with the probiotics. Zonulin is a protein of the haptoglobin family released from liver and intestinal epithelial cells and has been described as the main physiological modulator of intercellular tight junctions [133]. Increased zonulin concentrations are related to changes in tight junction competency and increased GI permeability [133]. The “leak” in the paracellular absorption route enables antigens to pass from the intestinal environment, challenging the immune system to produce an immune response and subsequent inflammation and oxidative stress [134,135,136]. Lamprecht et al. [63] suggested that the supplemented probiotics may activate the TLR2 signaling pathway resulting in improved intestinal barrier function, thus reducing an athlete’s susceptibility to endotoxemia and associated cytokine production [137].
• A small number of studies assessing GI benefit in athletes and physically active individuals have yielded mixed results with considerable variation in methodology, making comparison difficult.
• Positive results reported included decreases in concentrations of zonulin and endotoxin, intestinal hyperpermeability and duration of GI-symptom episodes.
Mechanism of action
Given that different strains and product formulations exist, explaining the mechanism of action becomes a rather complex task. An additional challenge in probiotic research is that a mechanism of action involving the gut microbiota is not confirmed, or even examined, in the majority of cases and there certainly are mechanisms outside of the GI tract systemically and in other microbiota niches. Clinical studies track probiotic “inputs” (whether a single strain or multiple strains) and health “outputs”, often without knowing what happens in between. This shortcoming further emphasizes the need to not use the general term probiotics, when describing mechanisms of action, but try to specify the strains [138]. This does not mean the mechanisms are the same for each strain, nor that precise mechanisms have been proven. For example, bacterial strains such as L. reuteri SD2112 (ATCC 55730) and L. reuteri RC-14 are different genetically and functionally, with the former producing reuterin believed to be important for inhibition of pathogens in the gut [139] and the latter producing biosurfactants that inhibit attachment of uropathogens [140]. Finally, several food products and dietary supplements may contain multiple species and strains in the same product. To fully explain the in-depth mechanisms of action is both out of the scope of this Position Statement and poorly understood in general. However, interested readers are directed to other resources [138, 141]. The question whether multi-strain or multi-species probiotics are better than single strain or single species probiotics depends on the outcome measure, dosage, and study population. Potential additive or even synergistic benefits would need to be validated in a control clinical study, and currently those data do not exist. Mechanisms of action in relation to the effects of probiotic supplementation in athletes has been less described [40]. Here we discuss support of the gut epithelial barrier, increased adhesion to intestinal mucosa, the effects of postbiotics, modulation of the immune system, and improved nutrient absorption.
Support of the gut epithelial barrier
The intestinal barrier is a major defense mechanism used to maintain epithelial integrity and protect the host from the environment. Defenses of the intestinal barrier consist of the mucous layer, antimicrobial peptides, secretory IgA and the epithelial junction adhesion complex [142]. Once this barrier function is disrupted, bacterial and food antigens can reach the submucosa and induce inflammatory responses [143, 144]. Consumption of non-pathogenic bacteria can contribute to intestinal barrier function, and probiotic bacteria have been extensively studied for their involvement in the maintenance of this barrier. However, the mechanisms by which probiotics enhance intestinal barrier function are not fully understood. Anderson et al. [145] indicated that enhancing the expression of genes involved in tight junction signaling is a possible mechanism to reinforce intestinal barrier integrity. Probiotics may promote mucous secretion as one mechanism to improve barrier function and the exclusion of pathogens. Several Lactobacillus species have been noted to increase mucin expression in human intestinal cell lines and, in the case of a damaged mucosa, may thus help restoration of the mucus layer. However, this protective effect is dependent on Lactobacillus adhesion to the cell monolayer, which likely does not occur in vivo [146, 147]. Therefore, mucous production may be increased by probiotics in vivo, but further studies are needed to make a conclusive statement.
Strenuous and prolonged exercise place stresses on the GI tract that increase the likelihood of discomfort, abdominal cramping, acid reflux (heartburn), nausea, vomiting, diarrhea, and permeability of the gut that may allow endotoxemia to occur [41]. Splanchnic hypoperfusion leading to ischemia in the gut is accepted as a principal cause, with additional contributions from nutritional, mechanical (e.g., jarring), and genetic influences that make some individuals more susceptible than others [41]. Probiotic support to increase resilience of the GI tract against ischemia is of interest to athletes, particularly for those involved in prolonged endurance events that have the greatest occurrence of GI problems that can impair or stop performance. Mechanistically, prolonged or strenuous exercise may increase key phosphorylation enzymes [148], disrupting tight junction proteins claudin (influenced by protein kinase A) and occludin (influenced by both protein kinase C and tyrosine kinase). Acute changes in tight junction permeability and paracellular transport may lead to a greater prevalence of systemic LPS. LPS from Gram-negative intestinal bacteria may provoke immune responses and endotoxin-associated symptoms characteristic of GI complaints often experienced in runners [148]. Despite this, research is relatively sparse on whether prolonged training or ultra-endurance events actually result in elevated LPS, particularly in more “recreationally active” athletes; or whether targeted nutrition strategies offer beneficial support. LPS translocation across the GI tract can provoke systemic immune reactions with varied consequences [149]. Specifically, LPS attachment to LPS-binding protein and its transference to an MD 2/TLR4/CD14 complex activates NF-κB and various inflammatory modulators (TNF-α, IL-1β, IL-6 and CRP). This sequence is considered a protective mechanism to minimize bacterial entry across the GI tract. Under normal physiological conditions, endotoxins from gram negative bacteria are usually contained locally, with only relatively small quantities entering the systemic circulation. However, when GI defenses are either disrupted (i.e., luminal damage from exercise) or LPS “sensing” is “overloaded”, a heightened inflammatory response may result which could, in part, relate to GI symptoms associated with exercise [150]. This effect could have implications for daily recovery strategies throughout prolonged training periods, and in the days following ultra-endurance events.
Roberts et al. [77] suggested a multi-strain pro/prebiotic intervention maintains tight junction stability. Further, studies have demonstrated that regular use of probiotics can improve epithelial resistance by establishing competitive “biofilm” formation. Indeed, as LPS types vary across Gram-negative bacteria species, some LPS are poorly sensed by TLR4 and may have more direct impact on NF-κB activation [151]. Therefore, prevention of LPS translocation through maintained epithelial integrity and/or increased preponderance of Gram-positive genera may offer potential therapeutic benefit [152]. Specifically, the provision of bacteria belonging to the Lactobacillus genus may work by activating TLR2 and hence produce more favorable innate immune responses [153, 154]. Supplementation with a multi-strain probiotic for 14 weeks decreased fecal zonulin levels, supporting improved tight junction stability through improved intestinal barrier integrity [63]. A mechanistic explanation for an improved intestinal barrier function after probiotic treatment is provided by Karczewski et al. [155], who postulate that certain lactic bacteria might activate the TLR2 signaling pathway. TLR2 is localized in the membranes of intestinal wall cells and from there communicates with microbial products from Gram-positive bacteria [115]. Furthermore, activation of the TLR2 signaling pathway can enhance epithelial resistance in vitro [156]. Therefore, supplemented probiotics may suppress bacteria that activate the zonulin system (e.g. Gram-negative bacteria), settle in the deep intestine, and activate the TLR2 signaling pathway.
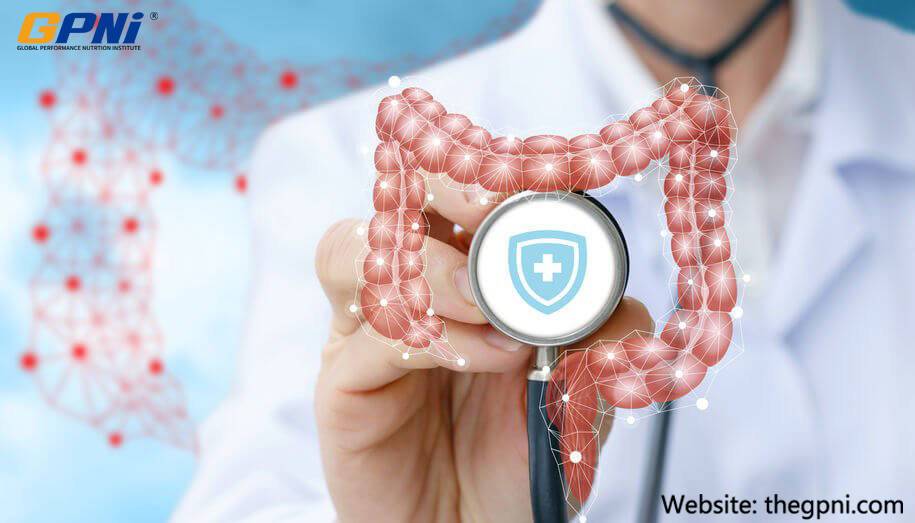
Adhesion to intestinal mucosa
“Competitive exclusion” is a term used to describe the vigorous competition of one species of bacteria for receptor sites in the intestinal tract over another species. The mechanisms used by one species of bacteria to exclude or reduce the growth of another species include: creation of a hostile microecology, elimination of available bacterial receptor sites, production and secretion of antimicrobial substances and selective metabolites, and competitive depletion of essential nutrients [141]. Adhesion of probiotics to the intestinal mucosa has been shown to favorably modulate the immune system [157, 158] and pathogen antagonism [159]. In addition, probiotics are able to initiate qualitative alterations in intestinal mucins that prevent pathogen binding [160] while some probiotic strains can also induce the release of small peptides or proteins (i.e., defensins) from epithelial cells [161]. These small peptides/proteins are active against bacteria, fungi and viruses [162] and may stabilize the gut barrier function [163]. Specific adhesiveness properties related to the interaction between surface proteins and mucins may inhibit the colonization of pathogenic bacteria and are a result of antagonistic activity by some strains of probiotics against adhesion of GI pathogens [164]. For example, lactobacilli and bifidobacteria can inhibit a broad range of pathogens, including E. coli, Salmonella, Helicobacter pylori, Listeria monocytogenes, and Rotavirus [165,166,167,168,169,170,171]. To gain a competitive advantage, bacteria can also modify their environment to make it less suitable for their competitors, such as producing antimicrobial substances (i.e., lactic and acetic acid) [172]. Some lactobacilli and bifidobacteria share carbohydrate-binding specificities with certain enteropathogens [173, 174], which makes it possible for the strains to compete with specific pathogens for the receptor sites on host cells [175]. In general, probiotic strains are able to inhibit the attachment of pathogenic bacteria by means of steric hindrance at enterocyte pathogen receptors [176].
Postbiotics
Postbiotics comprise metabolites and/or cell-wall components released by probiotics and offer physiological benefits to the host by providing additional bioactivity [4]. The potential benefits of these metabolites and/or cell wall components should not only be considered to be associated with probiotics but more generally to metabolites produced by bacteria during fermentation, including bile acid fermentation. Several compounds have been collected from several bacteria strains including SCFAs, enzymes, peptides, teichoic acids, peptidoglycan-derived muropeptides, endo- and exo-polysaccharides, cell surface proteins, vitamins, plasmalogens, and organic acids [177,178,179]. Despite the fact that the mechanisms implicated in the beneficial health effects of postbiotics are not fully elucidated, they possess different functional properties including, but not limited to, antimicrobial, antioxidant, and immune modulation [4]. These properties can positively affect the microbiota homeostasis and/or the host metabolic and signaling pathways, physiological, immunological, neuro-hormone biological, regulatory and metabolic reactions [180, 181].
In the majority of cases, postbiotics are derived from Lactobacillus and Bifidobacterium species; however, Streptococcus and Faecalibacterium species have also been reported as a source of postbiotics [177, 179]. SCFAs produced by the gut microbiota act as signaling molecules improving regulation of lipid metabolism, glucose homeostasis, and insulin sensitivity through the activation of receptors such as G protein-coupled receptors (GPRs) to regulate of energy balance while maintaining metabolic homoeostasis [182, 183]. Specific SCFAs (e.g. butyrate, acetate and propionate) also contribute to plasma cholesterol homeostasis in rodents and humans [184]. Some studies [185,186,187] determined that cell-free extracts from lactic acid bacteria exhibit higher antioxidant capacity than whole cell cultures, suggesting that the antioxidant capacity could be attributed to both enzymatic and non-enzymatic intracellular antioxidants.
Through postbiotic action, it seems plausible that probiotics can increase exercise performance as seen through a delay in fatigue in athletes by virtue of their production of SCFAs. In addition, species within the Lactobacillus genus synthesize lactic acid, which is converted to butyrate and later to acetyl-CoA, which is used in the Krebs Cycle to generate adenosine triphosphate (ATP). However, these processes occur mostly in the gut so whether or not this would impact skeletal muscle performance remains to be determined [188]. Another mechanism is by antioxidant action, which can attenuate muscle injury induced by reactive oxygen species, among others [92]. Antioxidant effects found in probiotics are linked to the synthesis of antioxidant substances such as vitamins B1, B5 and B6 [141]. Moreover, probiotic supplementation reduces the risk of developing hyperglycemia, a condition known to be linked to oxidative stress [189, 190]. Finally, the improvement in intestinal homeostasis, including the absorption process, may favor the absorption of antioxidants, increasing the availability of these substances [58].
One of the proposed mechanisms involved in the health benefits afforded by probiotics includes the formation of low molecular weight compounds (< 1000 Da), such as organic acids, and the production of antibacterial substances termed bacteriocins (> 1000 Da). Organic acids, in particular acetic acid and lactic acid, have a strong inhibitory effect against Gram-negative bacteria, and are considered the main antimicrobial compounds responsible for the inhibitory activity of probiotics against pathogens [191,192,193]. The undissociated form of the organic acid enters the bacterial cell and dissociates inside its cytoplasm. The eventual lowering of the intracellular pH or the intracellular accumulation of the ionized form of the organic acid can lead to the death of the pathogen [194].
Intestinal bacteria also produce a diverse array of health-promoting fatty acids. Certain strains of intestinal bifidobacteria and lactobacilli can produce conjugated linoleic acid (CLA), a potent anti-carcinogenic agent [195, 196]. An anti-obesity effect of CLA-producing L. plantarum has been observed in diet-induced obesity in mice [197]. Recently, the ability to modulate the fatty acid composition of the liver and adipose tissue of the host upon oral administration of CLA-producing bifidobacteria and lactobacilli has been demonstrated in a murine model [196]. Finally, certain probiotic bacteria are able to produce so-called de-conjugated bile acids, which are derivatives of bile salts. De-conjugated bile acids show a stronger antimicrobial activity compared to that of the bile salts synthesized by the host organism [141].
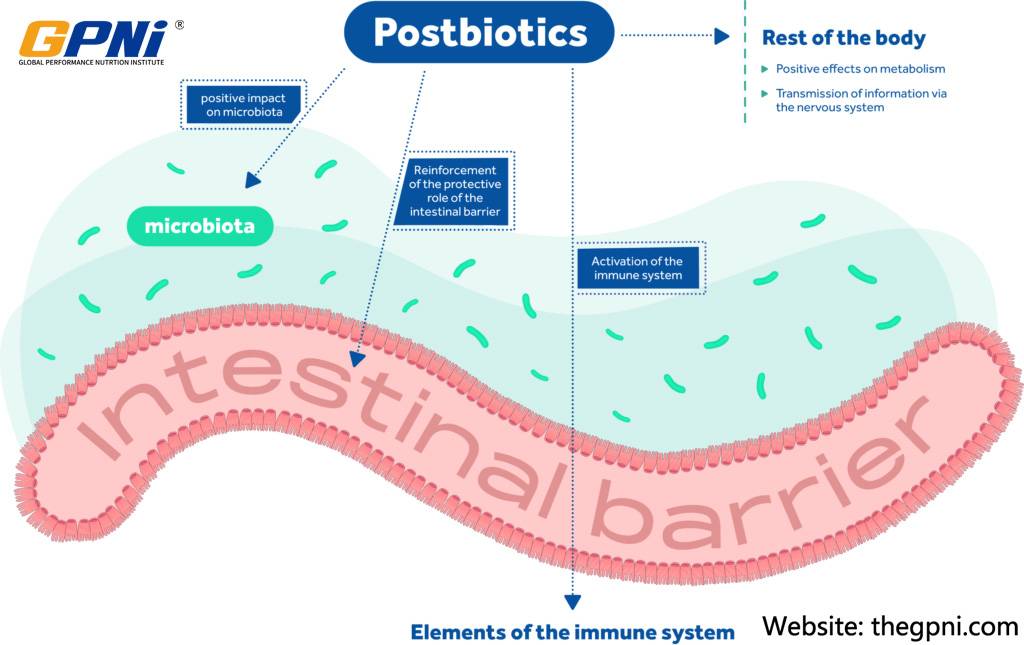
Modulation of the immune system
Numerous studies have shown that prolonged intense physical exercise is associated with a transient depression of immune function in athletes. While moderate exercise beneficially influences the immune system [198], a heavy schedule of training and competition can impair immunity and increase the risk of URTIs due to altered immune function [116, 199, 200]. Both innate immunity and acquired immunity are decreased following prolonged exercise [199,200,201]. It is well known that probiotic bacteria can exert an immunomodulatory effect; however, research from non-athletic populations may not be translatable to athletes. Further, the manipulation and control of the immune system by probiotics is difficult to evaluate and make general conclusions. However, several studies investigating the effects of probiotics in athletes have reported improvement in low-grade inflammation [42, 63], as well as increased resistance to URTIs [57, 60, 69, 78] and reduced duration of URTI [79].
Modulation of the immune system to increase defenses against URTIs currently is the most extensively researched area. The GI tract is a major gateway for pathogen entry, and as such, is heavily protected by the immune system. The immune system can be divided between the innate and adaptive systems. The adaptive (acquired) immune response depends on B and T lymphocytes, which are specific for particular antigens. In contrast, the innate immune system responds to common structures called pathogen-associated molecular patterns (PAMPs) shared by the vast majority of pathogens [202]. The primary response to pathogens is triggered by pattern recognition receptors (PRRs), which bind PAMPs. The best-studied PPRs are TLRs. In addition, extracellular C-type lectin receptors (CLRs) and intracellular nucleotide-binding oligomerization domain-containing protein NOD-like receptors are known to transmit signals upon interaction with bacteria [203]. It is well established that probiotics can suppress intestinal inflammation via the downregulation of TLR expression, secretion of metabolites that may inhibit TNF-α from entering blood mononuclear cells, and inhibition of NF-ĸB signaling in enterocytes [202].
Probiotics can enhance innate immunity (first-line-of-defense) by upregulating immunoglobulins, antimicrobial proteins, phagocytic activity, and natural killer cell activity, and enhance acquired immunity by improving antigen presentation and function of T and B lymphocytes to neutralize pathogens and virally-infected cells [10, 204]. These effects are of particular importance to athletes because exercise may increase susceptibility to URTIs by decreasing salivary IgA, decreasing cell-mediated immunity by decreasing type 1 T lymphocytes to make recurrent infections more likely, and increasing glucocorticoid suppression of monocyte/macrophage antigen presentation and T lymphocyte functions [205, 206]. The majority of placebo-controlled clinical trials assessing the efficacy of probiotics for reducing incidence, duration, and severity of URTI in athletes report beneficial outcomes. However, many different probiotics have been used and the differences in trial protocols and outcome measures complicate the drawing of more specific conclusions.
Improved nutrient absorption
Supplementation with some probiotic strains has been suggested to improve dietary protein absorption and utilization [207]. While not fully elucidated, several studies indicate a plausible role [208], yet a clear mechanism of action is lacking. As noted, probiotics can potentially improve intestinal barrier function by modulating tight junction permeability which may improve nutrient absorption.
Improving the digestibility of protein can speed recovery of strength after muscle-damaging exercise [209], and promote glycogen replenishment after exercise. B. coagulans produce digestive enzymes [97] active under gut conditions (alkaline proteases). These proteases can digest proteins more efficiently than the endogenous human proteases alone [96]. B. coagulans GBI-30, 6086 enhances the health of the cells of the gut lining improving nutrient absorption including minerals, peptides, and amino acids by decreasing inflammation and encouraging optimum development of the absorptive area of the villi [98].
In a computer-controlled in vitro model of the small intestine, B. coagulans GBI-30, 6086 enhanced amino acid absorption while improving colon health [208]. In recreationally-trained males, Jäger et al. [43] found the co-administration of B. coagulans GBI-30, 6086 and 20 g of protein improved recovery 24 and 72 h, and muscle soreness 72 h post-exercise. Furthermore, Toohey et al. [103], noted B. subtilis DE111 supplementation with a post-workout recovery drink containing 20 g of protein reduced body fat percentage after 10 weeks of resistance training compared with the same post-workout recovery drink and a placebo in female athletes. Toohey et al. [103] speculated improved amino acid uptake in the probiotic group may have resulted from more efficient protein digestion, simulating the effects of a higher daily protein intake.
Key Points 5 – Mechanisms of Action
• There are dozens of bacterial strains that can be considered as probiotics, particularly those that produce lactic acid. However, each strain is unique with respect to how it responds to and affects the host.
• The mechanisms underlying the beneficial effects of probiotics in athletes are largely unknown but are likely to be multifactorial.
• Consumption of some probiotic strains may improve intestinal barrier function by modulating tight junction permeability. However, the mechanisms by which probiotics enhance intestinal barrier function are not sufficiently studied.
• Adhesion of probiotics to the intestinal mucosa may be a mechanism for modulation of the immune system. Probiotics also cause alterations in intestinal mucins that prevent pathogen binding.
• Probiotics may support microbiota and postbiotic production which possess different functional properties including, but not limited to, antimicrobial, antioxidant, and immunomodulatory.
• Probiotics may enhance innate immunity by upregulating immunoglobulins, antimicrobial proteins, phagocytic activity, and natural killer cell activity, and also enhance acquired immunity by improving antigen presentation and function of T and B lymphocytes to neutralize pathogens and virally-infected cells.
• Probiotics can potentially modulate intestinal permeability and health of the cells of the gut lining improving nutrient absorption including minerals, peptides, and amino acids by decreasing inflammation and encouraging optimum development of the absorptive area of the villi.
Safety and health
The concept of probiotics is not new. Around 1900 Nobel laureate, Elie Metchnikoff, discovered that the consumption of live bacteria (L. bulgaricus) in yogurt or fermented milk improved some biological features of the GI tract [210]. Bacteria with claimed probiotic properties are now widely available in the form of foods such as dairy products and juices, and also as capsules, drops, and powders. Probiotics have been used safely in foods and dairy products for over a hundred years. Some of the most common commercially available strains belong to the Lactobacillus and Bifidobacterium genera. In this respect, well-studied probiotic species include Bifidobacterium (ssp. adolescentis, animalis, bifidum, breve, and longum) and Lactobacillus (ssp. acidophilus, casei, fermentum, gasseri, johnsonii, reuteri, paracasei, plantarum, rhamnosus, and salivarius) [211]. An international consensus statement in 2014 indicated that these are likely to provide general health benefits such as normalization of disturbed gut microbiota, regulation of intestinal transit, competitive exclusion of pathogens, and production of SCFAs [1].
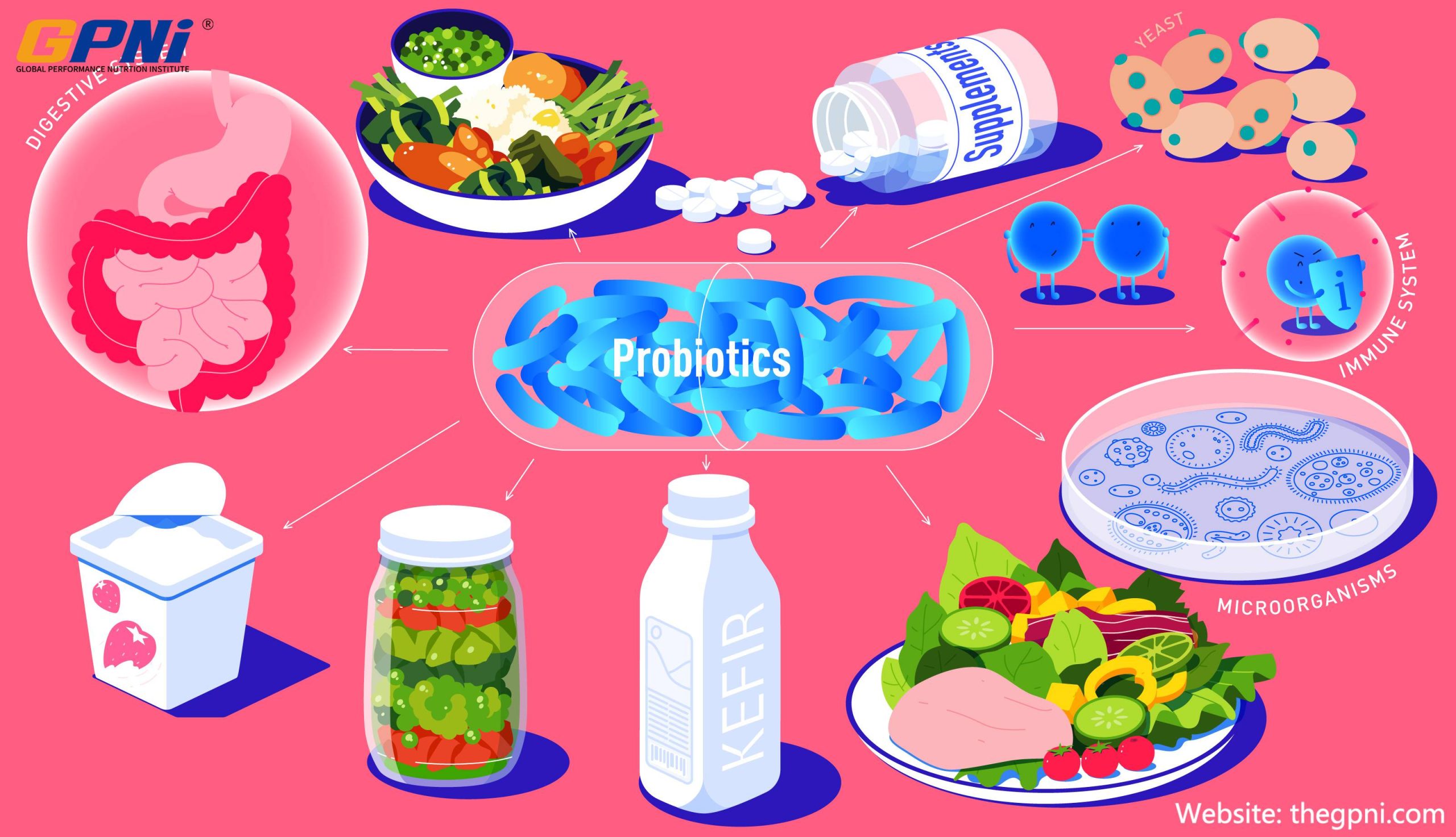
Beyond athletes and physically active individuals, there is a large body of preclinical and clinical research on the GI benefits of probiotics in healthy individuals and in a wide range of health conditions. These applications include treatment and prevention of acute diarrhea, prevention of antibiotic-associated diarrhea, treatment of hepatic encephalopathy, symptomatic relief in irritable bowel syndrome, and prevention of necrotizing enterocolitis in preterm infants [212]. Overall, probiotics have an excellent safety profile with a large majority of clinical trials involving probiotics not giving rise to major safety concerns [213]. Of the adverse events (AEs) commonly reported, Marteau [214] outlined four classes of possible side effects of probiotic use: systemic infections, detrimental metabolic effects, cytokine-mediated immunologic adverse events in susceptible individuals, and transfer of antibiotic resistance genes. Of these, particular concern relates to probiotics potential to create (not improve or treat) systemic infections [49, 64, 215]. Further, probiotics have been studied in vulnerable groups, including infants, patients with severe acute pancreatitis, inflammatory bowel diseases, liver diseases, HIV, and other conditions [213, 216,217,218] with even greater cause for concern with the small number of products that contain high concentrations of up to 450–900 billion live bacteria per dose [211]. Many of the studies reporting AEs (rarely serious AEs) either do not utilize the appropriate biological sampling and identification techniques or AEs are poorly reported.
Commercially available probiotic products can be divided into single-strain (defined as containing one strain of a well-defined microbial species) and multi-strain (containing more than one strain of the same species or genus). The term multispecies is also used for products that contain strains from more than one genus [211], for example a product with a L. acidophilus strain, a L. reuteri strain, and a B. longum strain. Treatment with probiotics may involve the consumption of large quantities of bacteria, so safety is a primary concern. There are two aspects to safety: establishing the adverse effect profile of specific single-strain and multi-strain supplements (i.e., the safety of the strain(s) per se), and ensuring that marketed supplements meet stringent quality standards to ensure the correct strains are present and the product is free of contamination [217].
Safety assessments should take into account the nature of the specific probiotic microbe, method of administration, level of exposure, health status of the recipients, and the physiological functions the microbes are intended to perform [213]. However, most probiotics in commercial use are derived from fermented foods with a long history of safe consumption, or from microbes that may colonize healthy humans [212]. All common probiotic species are considered safe for the general population by the European Food Safety Authority (EFSA), although this definition does not provide guidance on the increasing use of probiotics in people with medical conditions. Moreover the benefits of probiotics are not validated by EFSA, jeopardizing the use of the term probiotic without an approved claim with some exceptions such as in Italy, Czech Republic, and Bulgaria [211]. Going beyond history of safe use, since 2007 the EFSA lists species presumed safe for human consumption under the “Qualified Presumption of Safety” (QPS) concept. The approach is based on experience that for selected organisms there are no reasonable safety concerns for human health. The list regularly monitors the body of knowledge through extensive scientific literature review, applied to a wide array of micro-organisms added in the food-chain. The QPS list concerns consumption by the general healthy population and does not take into consideration potential risks for vulnerable populations and this is clearly mentioned. The U.S. Food and Drug Administration (FDA) classifies probiotics individually but has classified many as Generally Recognized As Safe (GRAS), safe for the use in foods and infant products [219].
A systematic literature review of probiotic safety published in 2014 reported that “the overwhelming existing evidence suggests that probiotics are safe” for the general population, and that critically ill patients, postoperative and hospitalized patients and immunocompromised patients were the most at-risk groups wherein AEs occurred [220]. The general consensus is that probiotic ingestion is safe [221, 222], with large doses well tolerated and failing to exhibit any toxicity [223]. Indeed, low CFU dosage and intervention periods between 2 weeks to 6 months are generally used within clinical research models [224, 225]. In this position stand, which reviews studies focused on probiotic supplementation in athletes and physically active individuals, 11 studies measured AEs and general supplementation tolerance, while 30 studies did not. Of the 11 studies, a general consensus was made to conclude that probiotic supplementation was generally well tolerated with a very low level of adverse health effects. There was one instance in which mild GI symptoms (5 episodes) were reported, including flatulence and stomach rumbles during supplementation with a multi-strain probiotic in 22 active individuals [66]. AEs are often not well recorded in nutritional studies in general and probiotics are no exception to this. Overall, from the current body of research probiotic supplementation for healthy athletes and physically active individuals appears safe. Caution is warranted for those with serious health conditions, such as severe acute pancreatitis, inflammatory bowel diseases, liver diseases, and HIV. In these instances, it is advised that the patient consult with their health care practitioner before supplementing. Another consideration is supplementing evidence-based dosages and keeping the probiotic properly stored. Unlike, other familiar sports supplements, probiotics are live organisms and may require specific storage requirements including refrigeration.
Key Points 6 – Safety and Health.
• Probiotics have been used safely in foods and dairy products for over a hundred years.
• Well-studied probiotic species include Bifidobacterium (ssp. adolescentis, animalis, bifidum, breve, and longum) and Lactobacillus (ssp. acidophilus, casei, fermentum, gasseri, johnsonii, reuteri, paracasei, plantarum, rhamnosus, and salivarius). • Well-studied probiotic species include Bifidobacterium (ssp. adolescentis, animalis, bifidum, breve, and longum) and Lactobacillus (ssp. acidophilus, casei, fermentum, gasseri, johnsonii, reuteri, paracasei, plantarum, rhamnosus, and salivarius).
• Safety assessments should take into account the nature of the probiotic microbe, method of administration, level of exposure, health status of the recipients, and the underlying physiological functions the microbes are intended to perform.
• Four classes of possible side effects are commonly reported from probiotic use in vulnerable patient groups: systemic infections, detrimental metabolic effects, cytokine-mediated immunologic adverse events in susceptible individuals, and transfer of antibiotic resistance genes.
• The current body of research of probiotic supplementation for healthy athletes and physically active individuals suggests that they are safe for use.
• Caution is warranted for those with serious health conditions. In these instances, patients should consult with their health care practitioner before supplementing.
• Consumers are advised to supplement with probiotics strains and products within evidence-based dosages.
Regulation
Currently there is no clear set of recommendation or guidelines on probiotic use for athletes. The current body of research has investigated a wide variety of species/strains, duration of use, and dosages with several different intended purposes (Table 4). The effects of probiotics are strain specific, and therefore, strain identity is important to link to a specific health effect as well as to enable accurate surveillance and epidemiological studies. Unfortunately, government regulatory organizations are highly varied across national borders and jurisdictions in regulation of probiotics, making uniform recommendations difficult.
Table 4 Dosage range in studies investigating the effect of specific probiotic genera in athletes and physically active individuals
Type
Dosage range
Lactobacillus (n = 35)
1 × 109 – 10 × 1010 CFU
Bifidobacterium (n = 18)
7 × 107–9.5 × 109 CFU
Streptococcus (n = 8)
5 × 109–4.5 × 1010 CFU
Bacillus (n = 5)
5 × 108 – 5 × 109 CFU
Multi- species/strain (n = 17)
2 × 109–4.5 × 1010 CFU
In 2001, the FAO/WHO held the Expert Consultation on Evaluation of Health and Nutritional Properties of Probiotics, to develop standardized guidelines for evaluating probiotics in food that could lead to the substantiation of health claims [226]. The proposed guidelines recommend: 1) identifying of the genus and species of the probiotic strain by using a combination of phenotypic and genotypic tests as clinical evidence suggesting that the health benefits of probiotics may be strain specific, 2) in vitro testing to delineate the mechanism of the probiotic effect, and 3) substantiating the clinical health benefit of probiotic agents with human trials. Additionally, safety assessment of the probiotic strain should at a minimum determine: 1) patterns of antimicrobial drug resistance, 2) metabolic activities, 3) side effects noted in humans during clinical trials and after marketing, 4) toxin production and hemolytic potential if the probiotic strain is known to possess those properties, and 5) lack of infectivity in animal studies [226].
Table 5 Approved Canadian Probiotics Claims for Sports Performance
The regulation of probiotics differs between countries as there is no universally agreed framework. For the most part, probiotics are categorized as food and dietary supplements because most are delivered by mouth as a food or supplement. For example, Health Canada has provided a Natural Health Product monograph that includes dosage form(s), use(s) or purpose(s) recommended as well as minimum quantities for L. johnsonii (La1/Lj1/NCC 533, an adjunct to physician-supervised antibiotic therapy in patients with H. pylori infections, 1.25 × 108 CFU) (all strains, 1 × 107 CFU), L. rhamnosus (GG, Management of acute infectious diarrhea, 6 × 109 CFU, management/risk reduction of antibiotic-associated diarrhea, 1 × 1010 CFU) (all strains, 1 × 107 CFU), and S. boulardii / S. cerevisiae (all strains, Risk reduction of antibiotic-associated diarrhea, 1 × 1010 CFU) (all strains, 1 × 107 CFU). The probiotic product monograph contains both bacteria and fungi which have been pre-approved for the use or purpose which allows claims; “source of probiotics”, “helps support intestinal/gastrointestinal health”, “could promote a favorable gut flora” with 1 × 107 CFU daily. The minimum daily dose is the sum of CFU per day provided by all live microorganisms that are present in the product, and not the minimum amount of CFU per day for each of the microorganisms. Further, a duration of use statement is not required, nor is there any guidance provided. Cautions include; “If you have fever, vomiting, bloody diarrhea, or severe abdominal pain, consult a health care practitioner prior to use” and “If symptoms of digestive disorders (e.g., diarrhea) occur, worsen and / or persist beyond 3 days, discontinue use and consult a health care practitioner.” [227]. In Canada, probiotics have two modes of sale on the market, Natural and Non-Prescription Health Products Directorate (NNHPD) and Food Directorate [3, 228]. Health Canada uses a pre-market approval process for non-food like applications such as capsules, tablets, softgels and powders which requires companies to acquire a Natural Product Number (NPN) prior to bringing to market [3]. Table 5 below details the current licensed products and claims specific to sport performance using probiotic strain(s) in or outside the pre-approved monograph. This list is open access through the Health Canada LCNHPD (Licensed Natural Health Products Database) which allows consumers and retailers the ability to review claims on packaging to approved claims by the NNHPD [229].
NPN
Probiotic Species Used (Strains if available) and Potency
Sport Specific Claims Supported by Research outside of monograph
80,080,307
B. breve BR03 5 Billion CFU
S. salivarius ssp. thermophilus FP4 5 Billion CFU
Helps maintain gastrointestinal health which may assist in normal recovery of performance following exercise.
80,077,863
B. coagulans GBI-30, 6086 1 Billion CFU
B. coagulans GBI-30, 6086 could be used to improve symptoms of delayed onset muscle soreness (DOMS) after exercise. B. coagulans GBI-30, 6086 helps maintain gastrointestinal health which may assist in a normal recovery of performance following exercise.
80,040,732
L. helveticus 400 million CFU
B. longum subsp. longum 600 million CFU
Helps maintain the health of the immune system following periods of physical stress.
80,064,384
L. helveticus 10 Billion CFU
Promotes gastrointestinal health in physically active adults
Helps reduce the incidence of cold-like symptoms in adults with exercise-induced stress
80,064,386
L. helveticus 10 Billion CFU × 2
Promotes GI health in physically active adults
Helps support immune defenses against winter infections in healthy adults and in those having weakened immunity due to intensive sports activities
Promotes GI health, immune health and general well-being in physically active adults (including sporty individuals like athletes)
Reduces symptoms with upper respiratory tract infections
Helps reduce incidence of cold-like symptoms in adults with exercise-induced stress
With 20 Billion CFU per day, this product helps support the first line of body’s immune defenses (IgA production), which may be associated with lowering URTI risk in physically active adults (such as competitive athletes)
80,050,736
B. animalis subsp. lactis 23 Billion CFU
B. animalis subsp. lactis 50 million CFU
B. animalis subsp. lactis 1 Billion CFU
B. bifidum 50 million CFU
B. longum subsp. infantis 100 million CFU
L. acidophilus 24.8 Billion CFU
L. acidophilus 1 Billion CFU
Reduces the risk of developing upper respiratory tract illness in physically active adults
Reduces the duration of URTI in physically active adults
80,064,494
B. animalis subsp. lactis BI-04 10 Billion CFU
B. animalis subsp. lactis Bi-07 10 Billion CFU
L. acidophilus NCFM 10 Billion CFU
L. paracasei LPC-37 10 Billion CFU
Helps reduce the risk of developing URTI in physically active adults
80,068,830
B. animalis subsp. lactis Bi-04 2 Billion CFU
Reduces the risk of developing URTI in physically active adults Reduces the duration of URTI in physically active adults
80,080,161
B. longum subsp. longum 320 million CFU
L. helveticus 2.68 billion CFU
L. helveticus 5 Biillion CFU
Promotes GI health, immune health and general well-being in physically active adults (including sporty individuals like athletes)
Reduces symptoms associated with upper-respiratory tract illness (URTI). Helps shorten the duration of URTI episodes
Helps reduce the incidence of cold-like symptoms in adults with exercise-induced stress
Helps support the first line of the body’s immune defenses (IgA production), which may be associated with lowering URTI risk in physically active adults (such as competitive athletes)
Helps support immune defenses against winter infections in healthy adults and in those having weakened immunity due to intensive sports activities
Helps to reduce gastrointestinal discomfort (e.g., abdominal pain, nausea, vomiting) in those experiencing mild to moderate stress resulting from life events (e.g., academic exams)
Helps to moderate general feelings of anxiety
Promotes a healthy mood balance
Helps to reduce stress-related gastrointestinal complications such as abdominal pain
80,089,514
B. bifidum 3 Billion CFU
L. helveticus 5 Billion CFU
Helps support immune defenses against winter infections in healthy adults and in those having weakened immunity due to intensive sports activities
Helps to alleviate gastro-intestinal (GI) disturbances like flatulence, constipation, bloating and abdominal cramps in healthy adults
Promotes GI health, immune health and general well-being in physically active adults (including sporty individuals like athletes)
Reduces symptoms associated with upper-respiratory tract illness (URTI)
Helps shorten the duration of URTI episodes
Helps reduce the incidence of cold-like symptoms in adults with exercise-induced stress
Helps support the first line of the body’s immune defenses (IgA production), which may be associated with lowering URTI risk in physically active adults (such as competitive athletes)
Helps reduce the incidence of cold-like symptoms in stressed adults
80,091,070
B. animalis subsp. lactis 2 Billion
L. acidophilus 1 Billion
L. acidophilus 3 Billion
L. plantarum 14 Billion
Reduces the risk of developing upper respiratory track illness in physically active adults
Reduces the duration of upper respiratory tract illness in physically active adults
80,091,070
B. animalis subsp. lactis 2 Billion
L. acidophilus 1 Billion
L. acidophilus 3 Billion
L. plantarum 14 Billion
Reduces the risk of developing upper respiratory track illness in physically active adults
Reduces the duration of upper respiratory tract illness in physically active adults
80,087,974
NPN
B. animalis subsp. lactis 2.81 Billion CFU
B. animalis subsp. lactis 1.47 Billion CFU
B. animalis subsp. lactis 810 million CFU
B. animalis subsp. lactis 530 million CFU
B. bifidum 28 million CFU
D-Glucose 13 mg
D-Xylose 13 mg
L-Arabinose 7 mg
L. acidophilus 630 million CFU
L. casei 610 million CFU
L. paracasei 690 million CFU
L. plantarum 890 million CFU
L. salivarius 560 million CFU
Xylooligosaccharides 631 mg
2 × 109–4.5 × 1010 CFUReduces the risk of developing upper respiratory track illness in physically active adults<br> Reduces the duration of upper respiratory tract illness in physically active adults
Japan is viewed by many to be a global market leader given that probiotics are available as both foods and drugs [230], and was the first global jurisdiction to implement a regulatory system for functional foods and nutraceuticals in 1991. Under Japanese regulations, probiotic products are in a distinct category of foods known as Foods for Specific Health Uses (FOSHU). For probiotic food products, efficacy claims are prohibited on the labeling. If claims are to be made about efficacy, one must obtain special permission from the Ministry of Health and Welfare (MHLW) for the product to be considered FOSHU, for which substantiation of efficacy and safety is a mandatory requirement [231]. In Brazil, probiotics are considered as functional foods, and considered to be different from food. But legislation asks for safety and efficacy demonstration of food products and hence all these products must be registered and approved by a health authority called National Health Surveillance Agency Brazil (ANVISA) [230].
In the European Union, probiotics and food supplements are regulated under the Food Products Directive and Regulation (regulation 178/2002/EC; directive 2000/13/EU). All health claims for probiotics have to be authorized by EFSA which has issued a list of microbial cultures that have a Qualified Presumption of Safety [232], meaning that they do not require safety assessments. The EFSA is also responsible for assessing health claims made for probiotic products. So far, EFSA has rejected all submitted health claims for probiotics. While rigorous scrutiny of product claims is apparent, there appears to be little regulation of the manufacturing process and almost no post-marketing regulatory follow-up [233].
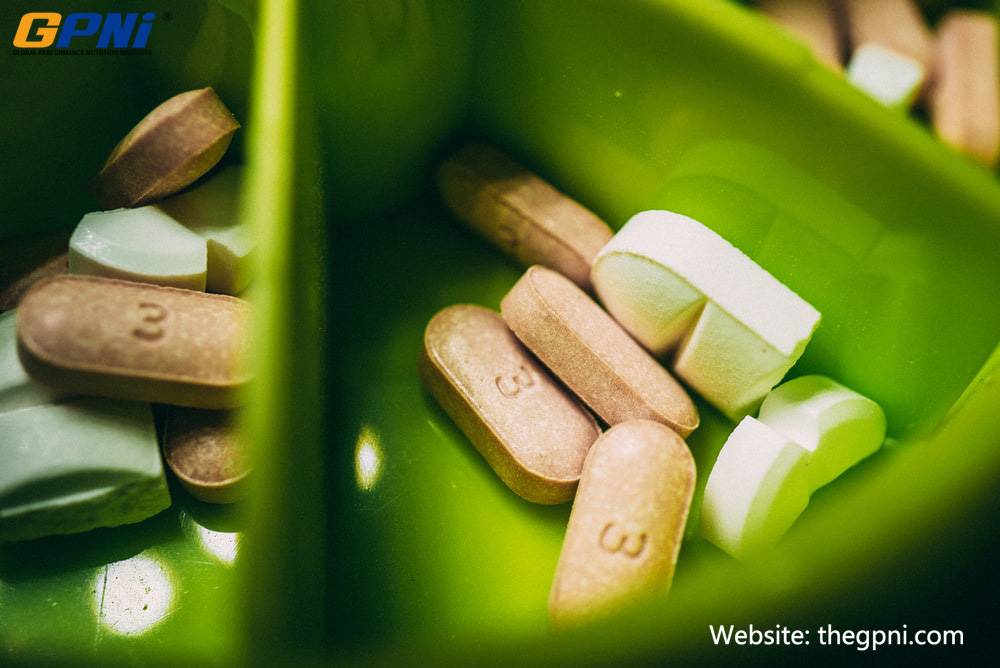
In the United States, government regulation of probiotics is complex. Depending on a probiotic product’s intended use, the FDA might regulate it as a dietary supplement, a food ingredient, or a drug. Many probiotics are sold as dietary supplements, which do not require FDA approval before they are marketed. Dietary supplement labels are permitted to make claims about how the product affects the structure or function of the body without FDA approval, but they cannot make health claims (claims that the product reduces the risk of a disease) without the FDA’s approval [234]. Further, dietary supplements are required to comply with Good Manufacturing Practice guidelines, but these do not extend to testing quality or efficacy [233]. From the examples provided, it is apparent that the current approach to regulation is inadequate and can lead to problems of quality, safety, and claim validity in commercial probiotic products used in a medical context, including those used in vulnerable populations [233].
In January 2017, the Council for Responsible Nutrition (CRN) and the International Probiotics Association (IPA) announced the development of scientifically-based best practices manufacturing guidelines for the labeling, storing, and stability testing of dietary supplements and functional foods containing probiotics [235]. These guidelines were designed to facilitate transparency and consistency in the probiotic sector. A key element of the guidelines is labelling probiotic products in CFU, the scientifically accepted unit of measure for probiotics and used to report probiotic quantity in many studies conducted to assess the safety or benefits of probiotics. Consistent with scientific literature, CFU are commonly used on probiotic product labels in many jurisdictions around the world to help consumers and healthcare professionals identify products providing probiotics in amounts shown to have benefit. However, United States regulations require dietary ingredients (with the exception of some vitamins) be labeled by weight. Labeling probiotic quantity by weight is not meaningful because this measure does not indicate the viability of the microorganisms in the product throughout shelf life. To the contrary, CFU are more representative of the quantity of viable microorganisms and gives consumers and healthcare professionals accurate information. The FDA has recently agreed that in addition to weight, probiotic amounts can also be labelled in CFU.
Upon examining the relevant literature investigating the effects of probiotic supplementation on athletes and those physically active, the genera commonly used included Lactobacillus (n = 35), Bifidobacterium (n = 18), Streptococcus (n = 8) and Bacillus (n = 5) (Table 3). In addition, several studies used a combination of species and strains (n = 17), ranging from two up to 14 different species/strains. The dose of probiotic administered is an important factor to be considered. In two reviews related to dietary supplementation in athletes, dosing regimens were reported in the range between 1 × 109 to 4 × 1010 CFU [10, 40]. In a 2018 consensus statement, the International Olympic Committee noted moderate support for probiotic use in athletes with a daily dose of 1 × 1010 live bacteria [5]. In our review, we report a wide range of doses (Table 4), and in several studies the dosage was not reported.
Similar to the type of probiotic used, the duration of supplementation has also been variable in the studies reviewed (Table 3). The shortest duration lasted 7 days [75, 76] and the longest lasted 150 days [68]. The duration and consistency of probiotic supplementation are important factors. Coqueiro et al. [188] noted that in clinical practice probiotic supplementation should be implemented for at least 14 days prior to competition or important events for the athlete. Therefore, studies that supplement for a similar or shorter period should be evaluated with caution. With the interruption of probiotic intake, there is a reduction in the microorganism administered in the colon, and with 8 days of supplementation discontinuation, the probiotic is no longer detectable in the gut [236]. Finally, there is some limited evidence that discrepancies exist between males and females, even after supplementation of probiotics with the same dose [61]. Future studies are needed in this area, with the intention of establishing a recommendation for each sex.
Key Points 7 Regulation
• No universally agreed upon framework exists for regulating commercial products containing probiotics across countries globally.
• Probiotic products should be labelled in CFU, the scientifically accepted unit of measure for probiotics and used to report probiotic quantity in many studies conducted to assess the safety or benefits of probiotics.
• Dosing regimens typically fall in range between 1 × 109 to 1 × 1011 CFU.
• The IOC noted moderate support for probiotic use when administered for several weeks in athletes with a daily dose of 1 × 1010 CFU.
• Genera of commonly used probiotics include Lactobacillus (n = 35), Bifidobacterium (n = 18), Streptococcus (n = 8) and Bacillus (n = 5).
• Single-strain and multi- species/strain products are commonly used, but combinations and individual dosing recommendations are not currently understood
• Males and females may respond to probiotic supplementation differently. Future research is needed in this area.
Future directions
Overall, the effects of probiotics in athletes have received less attention compared to animal studies and human clinical conditions in the general population. A PubMed search conducted in October 2019 yielded the following listings for various combinations of key terms: probiotic and athlete, n = 145; probiotic and rodent, n = 3407; probiotic and diabetes, n = 844; probiotic and child, n = 2930; probiotic and elderly, n = 2257. Clearly, the focus of the research community has been investigating the beneficial effects of probiotics on gut and immune health in various subgroups of the general population. In animals, probiotics have been associated with benefits including normalizing age-related drops in testosterone levels [237], increasing neurotransmitter synthesis [238], reducing stress-induced cortisol levels [239], reducing inflammation [100] and improving mood [240]. However, all these potential benefits lack current substantiation in human intervention trials in an athletic population. Here we discuss future research opportunities to explore in relation to the microbiome and athletes.
Body composition and muscle mass
It is well known that to increase levels of muscle mass, resistance training must be included in exercise regimens. Probiotic supplementation, both with and without resistance training, can decrease levels of body weight and fat mass in overweight and obese individuals, as well as female athletes [103, 241, 242]. Increases in fat free mass, however, have only been shown in animal models. Chen and colleagues [92] supplemented male Institute of Cancer Research (ICR) strain mice with L. plantarum TWK10 for 6 weeks. Mice were divided into three groups and daily doses of 0, 2.05 × 108, or 1.03 × 109 CFU were given to each group, respectively. The dosages chosen were modified from a comparable human dose equivalent to mouse body size. Relative muscle weight (%), as measured by combining the gastrocnemius and soleus muscles, were significantly increased in mice consuming the probiotic compared to placebo. Additionally, the number of type I fibers were increased significantly.
• No universally agreed upon framework exists for regulating commercial products containing probiotics across countries globally.
Mechanistically, it is plausible that Lactobacillus strains decrease levels of inflammation, thereby decreasing activation of intracellular proteins linked to muscle atrophy, which may eventually link to an observed increase in muscle mass. Chen et al. [92] also determined that probiotic supplementation increased forelimb grip strength and swim-to-exhaustion performance in mice, which may or may not have been related to improvement in muscle mass. Though improvements in body composition have been shown in humans, more studies examining decreased inflammation as a mechanism to increase muscle mass, in conjunction with reduction in fat mass, is warranted.
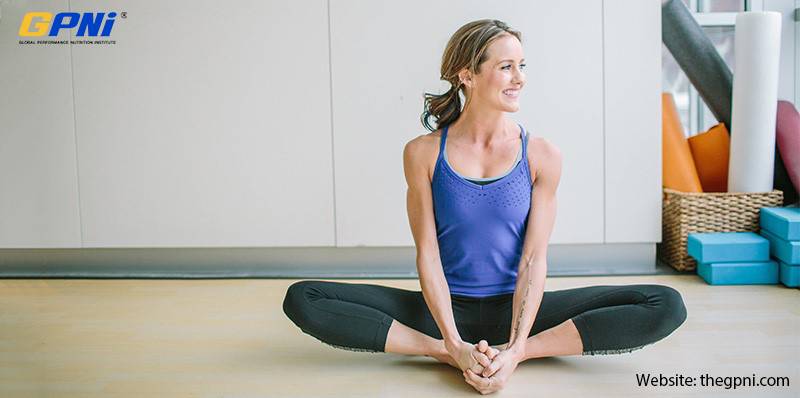
Buffering capacity in exercising muscles
Physiological fatigue, such as extreme fatigue after exercise, is accompanied by poor athletic performance and loss of favorable working conditions for tissues [243]. In response to higher intensity exercise, the concentration of lactate and hydrogen ions increased markedly resulting in an acidification in muscle and subsequent fatigue [244, 245]. Approximately 75% of the total amount of lactate produced is used for oxidative production of energy in the exercising body and can be utilized for the de novo synthesis of glucose in the liver [246].
Probiotic supplementation may have potential to remove and utilize blood lactate after exercise. For instance, most Lactobacillus species produce lactic acid, which could facilitate the production of butyrate by lactate-utilizing bacteria that first produce acetyl-CoA from lactate [247]. In the classical pathway, the enzymes phosphotransbutyrylase and butyrate kinase convert butyryl-CoA to butyrate and coenzyme A with concomitant formation of ATP. Thus, probiotics and the gut microbiota could play important roles in maintaining normal physiology and energy production during exercise. Several animal studies have been conducted with promising results. In mice who consumed a probiotic kefir daily over 4 weeks, swimming time-to-exhaustion was significantly longer, forelimb grip strength was higher and serum lactate, ammonia, blood urea nitrogen (BUN), and creatine kinase levels were lower after the swimming test [248]. In mice supplemented with L. plantarum TWK10 over 6 weeks, supplementation dose-dependently increased grip strength and endurance swimming time and decreased levels of serum lactate, ammonia, creatine kinase, and glucose after an acute exercise challenge [92]. Furthermore, the number of type I fibers in gastrocnemius muscle significantly increased with LP10 treatment. In a six-week human double-blind placebo-controlled clinical study, young healthy amateur runners supplemented with L. plantarum TWK10 and underwent an exhaustive treadmill exercise measurements and related biochemical indexes [85]. The TWK10 group had significantly higher endurance performance and glucose content in a maximal treadmill running test compared to the placebo group (P < 0.05), indicating that TWK10 supplementation may be beneficial to energy harvest. Together, these studies suggest a role in which certain probiotics may enhance energy harvesting, and have health-promotion, performance-improvement, and anti-fatigue effects. These are areas that may warrant further research consideration.
Considerations for future study designs
Several important methodological shortcomings in research design should be addressed to improve scientific evidence for the biological and clinical benefits of probiotics. For example, discrepancies between men and women, even after supplementation of probiotics with the same dose, are evident [61]. In this sense, in studies with both sexes, conflicting results may occur. In many instances and products, the recommendation for probiotic supplementation is no different for men and women, necessitating studies investigating this topic, with the intention of establishing a recommendation for each sex.
Other design concerns include the relatively small number of subjects, which may compromise the accuracy and interpretation of results. The period of supplementation is another important factor as the time of adaptation of the organism to the probiotic is approximately 14 days. Thus, studies that supplement for a similar or shorter period should be evaluated with caution. Further, with the interruption of probiotic intake, there is a reduction in the microorganism administered in the colon, and with 8 days of supplementation discontinuation, the probiotic is no longer detectable in the gut [236]. In clinical practice, it is common sense that probiotic supplementation should be implemented for at least 14 days prior to competition or important events for the athlete, given that during this period the GI tract adapts to the administered microorganism [188], and there may be mild, transient GI symptoms, such as flatulence [10]. The long-term effects of probiotic administration in athletes over several months or years on gut health, immune function and rates of illness are unclear, as in most studies the supplementation period was between 4 to 16 weeks.
Since many effects are dose-dependent, the amount of probiotic administered is an important factor to be considered. The range of oral probiotic supplementation is, approximately, 108–109 CFU per day, however, this value varies in each country [249, 250] and notably, no specific probiotic recommendation has been established for athletes or physical activity practitioners. Most of the studies do not control for previous levels of physical activity, so individuals within the same study may have very different levels of physical activity, making comparisons unrealistic. Finally, very few studies have evaluated the performance in strength exercises after supplementation with probiotics and this is an important area of sports and physical training to be studied.
Hormonal balance
Oral supplementation with selective bacteria holds promise in positively affecting the endocrine system. In mice, the microbiota can regulate testicular development and function [251], while androgen deficiency has substantially altered the microbiome [252]. Supplementation with a selenium-enriched probiotic in conjunction with a high-fat diet in male mice significantly alleviated the adverse effects of hyperlipidemia by reducing testicular tissue injury, increasing serum testosterone levels, and improving sperm indexes [253]. Further, aging mice supplemented with the probiotic bacterium L. reuteri had larger testicles and increased serum testosterone levels compared to their age-matched controls [237, 254].
In a human pilot study, supplementation with L. acidophilus and B. longum (1 × 109 CFU) did not alter plasma hormones, including testosterone, dihydrotestosterone, androstenedione, dehydroepiandrosterone sulfate, and sex hormone-binding globulin, in 31 healthy males (18 to 37 years old) over a two-month period [255]. However, another pilot study supplementing a probiotic and prebiotic (L. paracasei B21060 5 × 109 cells + arabinogalactan 1243 mg + fructooligosaccharides 700 mg + L-glutamine 500 mg) over 6 months in infertile male patients improved gonadal pathway function including increased follicle stimulating hormone, luteinizing hormone, and testosterone levels compared to a control group [256].
Interestingly, Tremellen et al. [257] proposed that gut-derived endotoxin can reduce gonadal function in obese males. Obesity and a high fat/high calorie diet can alter gut bacteria and intestinal wall permeability, leading to the passage of LPS from within the gut lumen into the circulation (metabolic endotoxemia), where it initiates systemic inflammation [258]. Endotoxin can reduce testosterone production by the testes, both by direct inhibition of Leydig cell steroidogenic pathways and indirectly by reducing pituitary luteinizing hormone drive and sperm production [259]. Tremellen and colleagues [257] theorized the male reproductive axis has evolved the capacity to lower testosterone production during times of infection and resulting endotoxin exposure, decreasing the immunosuppressive influence of testosterone, in turn enhancing the ability to fight infection. Weight loss and physical activity seem to improve these symptoms [260]. These novel findings suggest a potential impact for microbe therapy in obese and/or aging athletes by imparting hormonal and gonadal features of reproductive fitness typical of much younger healthy individuals. However, studies are severely lacking. In the future, larger sample sizes and more robust study designs will be needed.
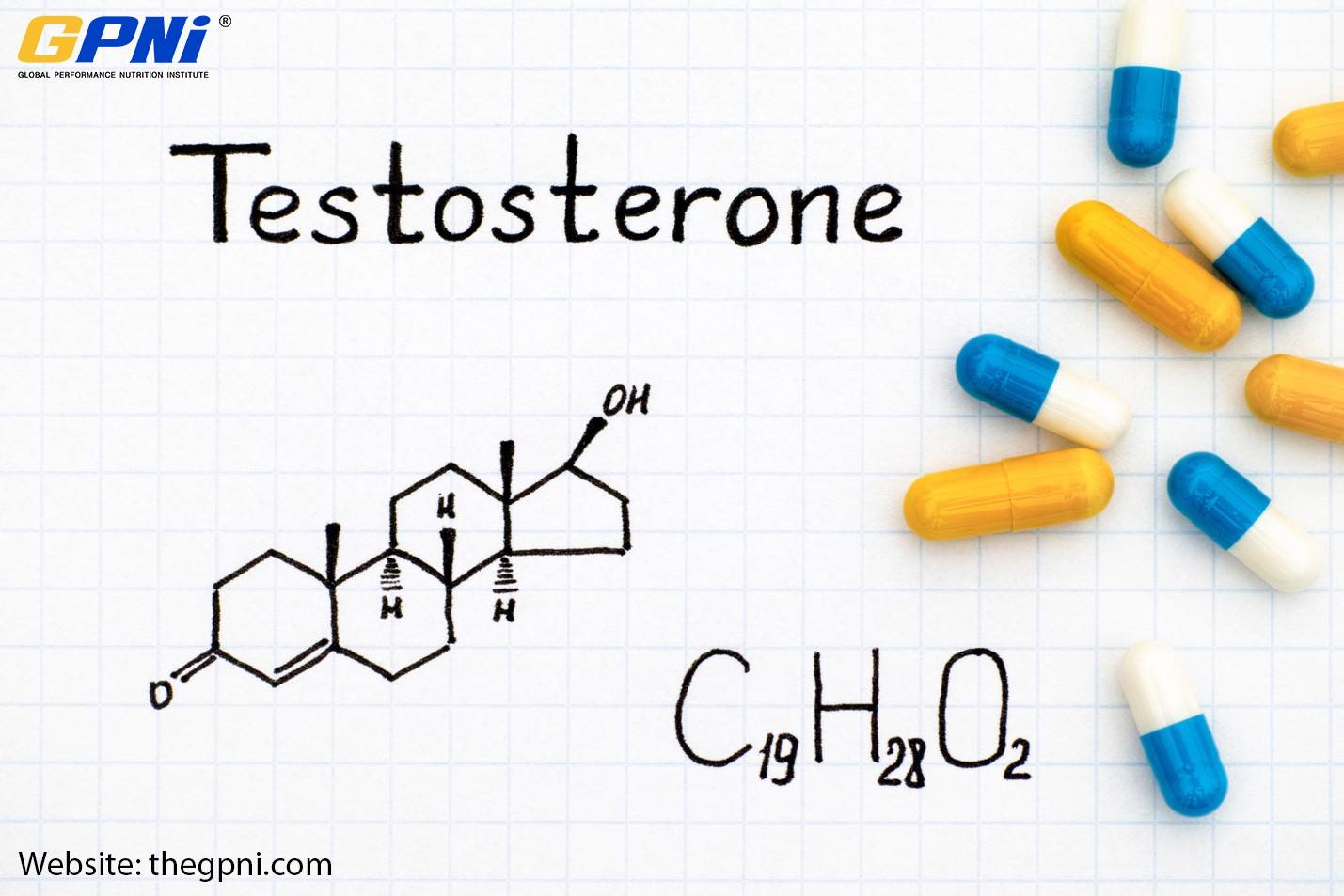
Inactivated “probiotics”
There is an increasing interest in supplementation with non-viable microorganisms or microbial cell extracts. By definition, probiotics are required to be alive, therefore inactivated microorganisms cannot be classified as such. However, preparations from certain probiotic species and strains (such as those from lactobacilli and bifidobacteria) have shown to maintain health benefits even after no longer being viable [261,262,263]. Inactivation can be achieved by different methods, including heat, chemicals (e.g., formalin), gamma or ultraviolet rays, and sonication, with heat treatment being the method of choice in most cases [228, 264, 265]. Importantly, these methods of inactivation may affect structural components of the cell differently, and therefore their biological activities [264, 265]. Piqué et al. (2019) suggested the presence of key structures in the cell or supernatant fractions may confer probiotic properties, mainly through immune-modulation, protection against pathogens, and fortifying the mucosal barrier integrity [261]. These different bacterial components include lipoteichoic acids, peptidoglycans, and/or exopolysaccharides [261].
Favorable properties of heat-killed bacteria have been observed in vitro [266], in animal models [264], and human trials [267, 268]. For example, in healthy subjects with high levels of self-reported psychological stress, supplementation with heat-killed L. plantarum L-137 significantly lowered incidence of URTI after 12 weeks compared to the control group [269]. This finding may have resulted from innate immunity stimulation as heat-killed L. plantarum L-137 has been reported to enhance type I IFN production in humans [270]. In athletes, there have only been two studies published examining the effect of these inactivated “probiotics”. In a randomized, double blind, placebo-controlled trial, 51 male athletes engaged in high intensity exercise (> 11 h per week) and consumed a placebo or heat-killed L. lactis JCM 5805 daily for 13 days [262]. Compared to placebo, supplementation increased the maturation marker of plasmacytoid DC pDC (CD86), responsible for the antiviral response, and decreased the cumulative days of URTI symptoms. Furthermore, ingestion decreased cumulative days of self-reported fatigue. In a longer duration randomized, double blind, placebo-controlled study, 49 long-distance runners consumed heat-inactivated L. gasseri CP2305 or placebo daily for 12 weeks [271]. No significant difference in physical performance between the CP2305 and placebo group were detected. However, CP2305 supplementation improved recovery from fatigue and relieved anxiety and depressive mood compared with placebo intake. Further, CP2305 intake prevented training-induced reduction of hemoglobin and facilitated exercise-induced increase in serum growth hormone levels. Moreover, gene expression profiling of peripheral blood leukocytes indicated that CP2305 prevented the stress-induced changes in the expression of genes related to mitochondrial functions. In relation to the gut microbiota, CP2305 intake increased the alpha- and beta-diversity, and the compositions of Bifidobacterium and Faecalibacterium. These compositional changes in the gut microbiota may have contributed to the recovery of fatigue and moderation of stress and anxiety through the gut-brain axis. Indeed, inactivated CP2305 can relieve stress in healthy young adults facing stressful conditions [272]. While encouraging, it is unclear how the daily intake of the heat-inactivated probiotics could affect the gut-brain axis and alter stress responses. Further research investigating potential mechanisms as well as more extensive studies with a wider range of athletes and exercise loads should be conducted. In addition, primary aims related to GI tract health and exercise performance should be more thoroughly assessed.
Mood and cognition
Physical health and mental health are strongly linked with depression, which is recognized as a leading cause of disability throughout the world [273]. Recently, it has been reported that 35% of individuals with depression also have symptoms of a leaky gut [274], which strengthens the notion of a link between the brain and the GI tract. As reported by Clarke et al. [275], gut bacteria contribute to various mood states in an individual. The gut-brain axis is a bidirectional pathway via the neural, endocrine, and immune systems. The mechanisms by which probiotics improve symptoms of depression and other mood disorders are via anti-inflammatory actions that reduce activity of the hypothalamic-pituitary-adrenal (HPA) axis [276].
Probiotics may be an effective treatment strategy for depression and mood disorders such as anxiety given the link between GI tract bacteria and the brain (i.e. the gut-brain axis), as decreased intestinal dysbiosis may have beneficial effects on mood. Only a few studies have been completed in human subjects that have examined the impact of probiotic supplementation on mood and anxiety. Benton and colleagues [210] reported that 3 weeks of supplementation with 1 × 108 CFU of L. casei had positive effects on mood, with subjects feeling increased clear-headedness, confidence, and elation compared to baseline. A study by Rao et al. [277], reported that 8 weeks of 8 × 107 CFU of L. casei given to individuals with chronic fatigue syndrome reduced anxiety symptoms. Similarly, Messaoudi and others [278] found decreased anxiety related behaviors after 2 weeks of a combination of L. helveticus and B. longum in 25 healthy adults. Moreover, 6 weeks supplementation of 4 × 109 CFU/live cells of L. fermementum LF16, L. rhamnosus LR06, L. plantarum LP01, and B. longum BL04 improved mood and sleep quality with a reduction in depressive mood state, anger and fatigue [279].
Overall, research on probiotics and mood in athletic populations is lacking. One review, completed by Clark and Mach [280] likened the psychological demands of exercise to physical stress. These authors concluded that the gut microbiota acts as an endocrine organ, secreting neurotransmitters such as serotonin and dopamine, thereby controlling the hypothalamic-pituitary axis in athletes. It is unclear whether these conclusions are attributable to the physiological or psychological stress, and more research is needed to expand on the current findings.
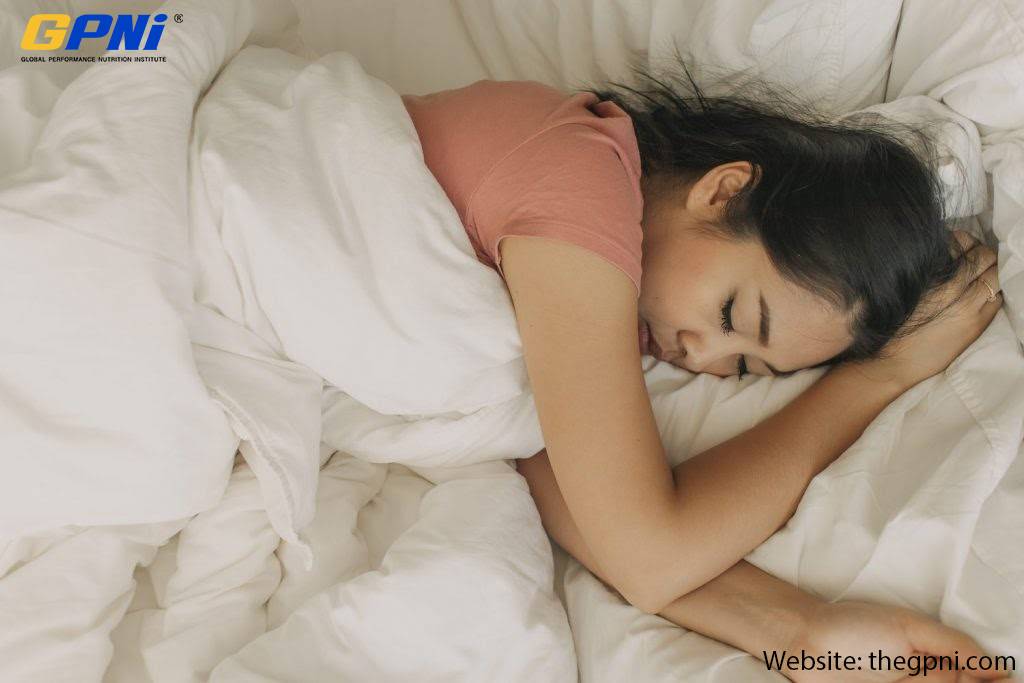
Muscle damage and recovery
Inflammation has been implicated in probiotic supplementation impacting body fat levels in overweight and obese individuals, as well as athletic populations. Research in this area, however, has been completed entirely in animal models. Zhao et al. [281] reported that supplementation of Akkermancia muciniphila in lean mice fed a chow diet for 5 weeks significantly improved markers of low-grade, chronic inflammation via measurement of LPS, and alleviated gains in both body weight and fat mass. Probiotic supplementation also increased anti-inflammatory factors α-tocopherol and β-sitosterol. Interaction between A. muciniphila and inflammatory processes may subsequently impact metabolic health and consequently body composition regulation. In humans, low-grade, chronic inflammation is a marker of many disease states and aspects of the metabolic syndrome. To date, no such research has been completed in athletic populations to clarify the impact of probiotic supplementation on body composition in athletes.
Neurotransmitter synthesis and release
Choline and its derivatives serve as components of structural lipoproteins, blood and membrane lipids, and as a precursor of the neurotransmitter, acetylcholine [282]. Choline is converted into acetylcholine via the enzyme choline acetyltransferase. Increasing plasma levels of choline could improve the production of acetylcholine, increase muscular contraction, and possibly delay fatigue in endurance exercise [282]. Elevated choline levels were observed in plasma of mice supplemented with L. rhamnosus compared to those fed with L. paracasei and controls [283]. In humans, probiotics and choline have been studied in the context of Trimethylamine N-oxide (TMAO). TMAO is an atherogenic metabolite that requires gut microbes for its generation through a metaorganismal pathway that begins with dietary consumption of trimethylamine (TMA) containing precursors such as choline, carnitine and phosphatidylcholine [284]. In a two-week clinical study on 19 healthy, non-obese males, supplementing with a multi-strain probiotic following a hypercaloric, high-fat diet failed to elevate plasma choline levels [285]. In a three-month pilot study investigating the effects of probiotic supplementation on TMAO plasma levels in hemodialysis patients, choline did not change compared to control group [286]. There is currently no research in athletes or active individuals, yet increases in plasma choline could (in theory) support increases in acetylcholine and consequently power, and endurance.
Nutrient timing
As indicated previously, various supplementation protocols have been implemented regarding probiotic consumption supplementation, including taking on an empty stomach, with food, and even after exercise. In relation, little is known pertaining to the optimal timing of probiotic intake for improved microbial survival and nutrient absorption. Tompkins et al. utilized an in vitro digestive model of the upper GI tract to investigate the timing effects of probiotic intake utilizing a multi-species encapsulated product containing L. helveticus R0052, L. rhamnosus R0011, B. longum R0175, and S. cerevisiae boulardii [287]. Results of this investigation showed that when a probiotic was consumed 30 min before a meal or with a meal, the bacteria survived in high numbers. Conversely, when the probiotic was taken 30 min after a meal, the bacteria did not survive in high numbers. Additionally, this study reported that consumption of the probiotic with 1% milk and oatmeal-milk gruel allowed for higher bacteria survival than when consumed with apple juice or spring water. Thus, future work should focus on the most favorable time to consume probiotics to promote survival in humans along with optimal nutrient/foodstuffs co-ingestion.
Response to a physical or mental stressor
Cortisol is a steroid hormone released by the adrenal glands in response to stress and increased levels have been related to suppression of the immune system in athletes [288,289,290]. Moreover, a connection has been established between the digestive tract and stress [291, 292]. Several studies that supplemented healthy young college students during exam preparation with probiotics (L. plantarum 299v and L. casei Shirota) reported attenuation of cortisol compared to a control group [293,294,295]. However, in an eight-week crossover design, 29 healthy male volunteers who supplemented with L. rhamnosus exhibited little difference in stress-related measures, HPA axis response, inflammation, or cognitive performance in comparison to placebo [296]. More recently, a systematic review and meta-analysis of clinical and pre-clinical literature on the effects of probiotics on anxiety asserted that probiotics may help reduce anxiety [297]. However, these findings have not yet been fully translated in clinical research in humans. More relevant to performance, eight endurance-trained males in a blinded randomized crossover design who supplemented with a probiotic beverage (L. casei, 1 × 1011 CFU) for seven consecutive days before a two-hour running exercise at 60% VO2max in hot ambient conditions (34.0 °C and 32% RH) failed to exhibit a decrease in cortisol response compared to a placebo [75].
Key Points 8 – Future Directions
• Probiotic therapy has the potential to positively affect the endocrine system (testosterone production), especially for obese and/or aging athletes.
• Modulation of the gut microbiome could alter the production/level of important neurotransmitters related to athletic performance.
• Probiotic supplementation may have an impact on stress; however, current research is limited.
• Preliminary animal research suggests probiotic supplementation may support the removal and utilization of blood lactate.
• Important methodological considerations must be addressed systematically in future research including the effect of: sex, sample size, duration, dose (type and amount), level of physical activity, and type of exercise.
Summary
Understanding whether probiotic supplementation plays a role in athletic performance is of interest to athletes who work to improve their training and competition performance. Moreover, this knowledge may be of general benefit to human health. Further studies are required to understand how the microbiome influences anti-inflammatory effects, optimal breakdown and utilization of consumed food, and other beneficial effects for overall health in athletes. Overall, the studies reviewed in this position statement provide modest evidence that probiotics can provide some clinical benefits in athletes and other highly active individuals (Table 3). The difficulty in interpreting the studies is illustrated by variations in clinical outcome measures and most importantly, as probiotic benefits are strain-specific, by different strains used in these studies.
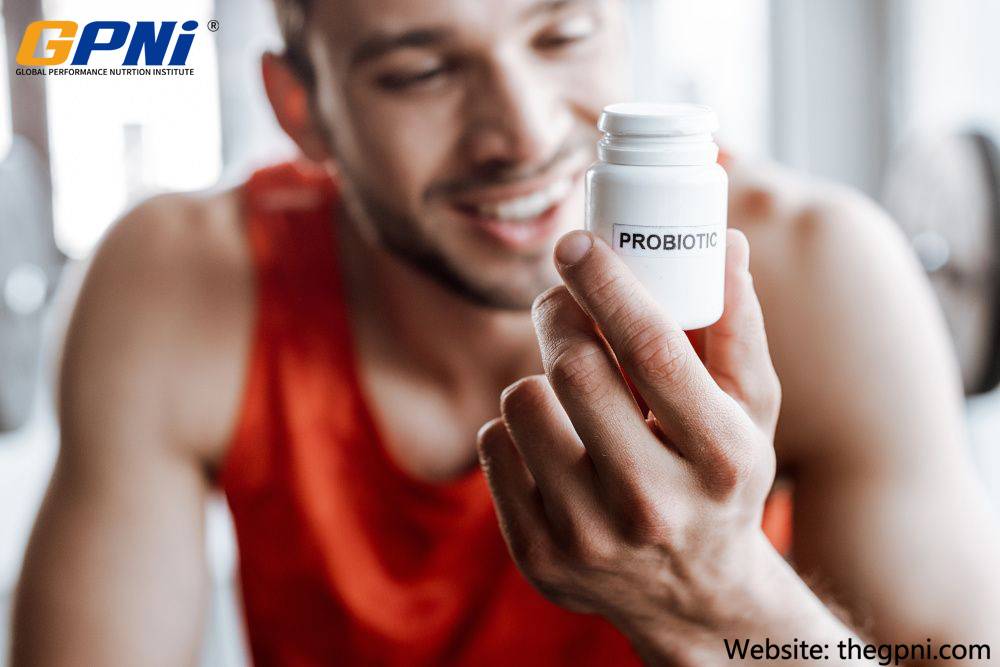
As outlined in Table 3, the following probiotic strains/species have been linked to an increase in athletic performance and/or recovery:
1、B. coagulans GBI-30, 6086 (BC30) at 1 × 109 CFU has beneficial effects in combination with protein on exercise recovery;
2、Encapsulated B. breve BR03 in combination with S. thermophilus FP4 at 5 × 109 CFU each has beneficial effects on exercise recovery and performance following muscle-damaging exercise;
3、L. delbrueckii ssp. bulgaricus at 1 × 105 CFU can increase VO2max and aerobic power;
4、L. acidophilus SPP, L. delbrueckii bulgaricus, B. bifidum, and S. salivarus thermophilus at 4 × 1010 CFU administered in form of a yogurt drink can increase VO2max;
5、L. plantarum TWK10 at 1 × 1010 CFU has been shown to increase endurance performance;
6、L. acidophilus, L. rhamnosus, L. casei, L. plantarum, L. fermentum, B. lactis, B. breve, B. bifidum and S. thermophilus at 4.5 × 1010 CFU can increase run time to fatigue in the heat.
The following probiotic strains/species have been linked to improved gut health in athletes (see Table 3):
1、L. rhamnosus GG at 4 × 1010 CFU in form of a milk-based drink,
2、B. bifidum W23, B. lactis W51, E. faecium W54, L. acidophilus W22, L. brevis W63, and L. lactis W58, at 1 × 1010 CFU;
3、L. salivarius (UCC118) (unknown dose).
The following strains/species have been shown to improve immune health in athletes, reducing the episodes, severity or duration of exercise-induced infections:
1、1.2 × 1010 CFU L. fermentum VRI-003 (PCC) at 1.2 × 1010 CFU and at 1 × 109 CFU in males;
2、L. casei Shirota (LcS) at 6.5 × 109 CFU twice daily;
3、L. delbrueckii bulgaricus, B. bifidum, and S. salivarus thermophilus at 4 × 1010 CFU administered in the form of a yogurt drink;
4、B. animalis subsp. lactis BI-04 2 × 1010 CFU;
5、L. gasseri 2.6 × 109 CFU, B. bifidum 0.2 × 109, and B. longum 0.2 × 109 CFU;
6、B. bifidum W23, B. lactis W51, E. faecium W54, L. acidophilus W22, L. brevis W63, L. lactis W58 at 1 × 1010 CFU;
7、L. helveticus Lafti L10 at 2 × 1010 CFU.
Given the small number of studies, and substantial variation in experimental approaches, dependent measures, and outcomes, more well-designed studies of probiotic supplementation in various athlete groups are warranted. While a majority of probiotics currently on the market, and tested in humans, feature the Lactobacillus, Bifidobacterium, and Bacillus genera, new microbiome research and technological advances are identifying potential next-generation probiotic candidates. Further research is needed not only to identify these discoveries, and validate their performance and recovery benefits in clinical settings.
Recommendations
Athletes and physically active individuals should thoroughly review health care and consumer information on specific applications, dosage, and possible contraindications of probiotic supplementation. As with any dietary supplementation, probiotics should be considered in the overall context of balanced dietary intake, i.e. nutrient needs should be met by a “food first” approach via consumption of whole foods rather than supplements. For example, recommending dietary supplements to developing athletes might overemphasize their importance in comparison to other training and dietary strategies [298]. In this context, it is also important to remember that some food-based probiotic products (e.g. yogurt) contain energy, carbohydrate, protein, and other nutrients that can form part of an athlete’s overall nutrition plan. Only reputable sources of commercially available supplements should be used to reduce the risk of contaminants that might contravene doping in sport regulations [5]. Athletes should be educated on the likely risks of contamination given that the World Anti-Doping Agency enforces a principle of strict liability for positive test results involving banned substances. Different formulations of probiotics from tablets or capsules to powder (added to drinks) or probiotic-enriched chewable tablets are available to meet individual preferences.
Probiotic supplements should be packaged, stored, handled, and transported in an appropriate manner. Athletes should take particular care in warm to hot environments and avoid, where possible, leaving supplements outdoors for long periods in direct sunlight, in a motor vehicle, or near an oven or other heat-generating appliances. New technology has led to probiotic supplements that do not require refrigeration, which may be ideal for athletes during travel. Supplements should also be kept dry at all times. During travel it might be useful for individuals to keep probiotics with other nutritional supplies, supplements, ergogenic acids or medications, or held by team personnel as required.
In terms of implementation, probiotic supplementation should commence at least 14 days before a major training period or competition to allow adequate time for transient colonization or adaptation period of bacterial species in the gut. Another important issue is the increased risk of GI problems during travel [299]. Supplementation with probiotics for individuals and athletes traveling could be included in an overall illness prevention plan. Tolerance and side effects should be monitored by the athlete, coach, and support staff and a medical opinion sought if there is ongoing concern. It is not unusual to experience transient increased activity in the gut during the colonization period (e.g., intestinal rumbling, increased flatulence, etc.) and athletes should be informed that mild side effects for a few days are not uncommon [61]. Athletes should be encouraged to review and monitor probiotic consumption on a daily basis to promote compliance and best practice usage. Compliance might be improved by having athletes take the probiotic supplement at the same time each day (e.g., at breakfast). Probiotic supplementation should be tested during the offseason or preseason phases, so the athlete is familiar with taking the probiotic supplements or foods before travel or major competition, and can see how he/she responds. This practice is also useful in the context of assessing individual tolerance and potential adverse effects.
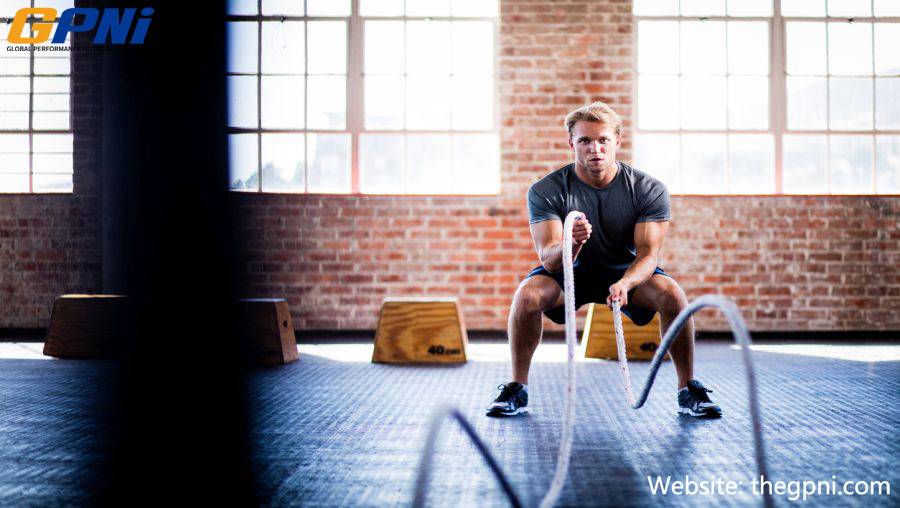
Position of the International Society of Sports Nutrition (ISSN)
After reviewing the scientific and medical literature in this area, the International Society of Sports Nutrition concludes the following in terms of probiotic supplementation as the official Position of the Society:
1、Probiotics are live microorganisms that, when administered in adequate amounts, confer a health benefit on the host (FAO/WHO).
2、Probiotic administration has been linked to a multitude of health benefits, with gut and immune health being the most researched applications.
3、Despite the existence of shared, core mechanisms for probiotic function, health benefits of probiotics are strain- and dose-dependent.
4、Athletes have varying gut microbiota compositions that appear to reflect the activity level of the host in comparison to sedentary people, with the differences linked primarily to the volume of exercise and amount of protein consumption. Whether differences in gut microbiota composition affect probiotic efficacy is unknown.
5、The main function of the gut is to digest food and absorb nutrients. In athletic populations, certain probiotics strains can increase absorption of key nutrients such as amino acids from protein, and affect the pharmacology and physiological properties of multiple food components.
6、Immune depression in athletes worsens with excessive training load, psychological stress, disturbed sleep, and environmental extremes, all of which can contribute to an increased risk of respiratory tract infections. In certain situations, including exposure to crowds, foreign travel and poor hygiene at home, and training or competition venues, athletes’ exposure to pathogens may be elevated leading to increased rates of infections. Approximately 70% of the immune system is located in the gut and probiotic supplementation has been shown to promote a healthy immune response. In an athletic population, specific probiotic strains can reduce the number of episodes, severity and duration of upper respiratory tract infections.
7、Intense, prolonged exercise, especially in the heat, has been shown to increase gut permeability which potentially can result in systemic toxemia. Specific probiotic strains can improve the integrity of the gut-barrier function in athletes.
8、Administration of selected anti-inflammatory probiotic strains have been linked to improved recovery from muscle-damaging exercise.
9、The minimal effective dose and method of administration (potency per serving, single vs. split dose, delivery form) of a specific probiotic strain depends on validation studies for this particular strain. Products that contain probiotics must include the genus, species, and strain of each live microorganism on its label as well as the total estimated quantity of each probiotic strain at the end of the product’s shelf life, as measured by colony forming units (CFU) or live cells.
10、Preclinical and early human research has shown potential probiotic benefits relevant to an athletic population that include improved body composition and lean body mass, normalizing age-related declines in testosterone levels, reductions in cortisol levels indicating improved responses to a physical or mental stressor, reduction of exercise-induced lactate, and increased neurotransmitter synthesis, cognition and mood. However, these potential benefits require validation in more rigorous human studies and in an athletic population.
Conclusion
Given all the known benefits and favorable safety profile of probiotic supplementation reported in the scientific and medical literature, probiotics are commonly used to optimize the health of athletes. Regular consumption of specific probiotic strains may assist with immune function and may reduce the number of sick days an athlete experiences when training or during competition. Certain probiotic strains may reduce the severity of respiratory infection and GI disturbance when they occur. Probiotic benefits are strain specific and dose dependent, and include improved gut-barrier function, nutrient absorption, recovery and performance in athletes. When choosing a probiotic product, athletes are encouraged to use clinically researched strains with validated benefits, matching the athletes desired health benefit. Studies investigating the effects of probiotics in athletic populations and on sports performance are limited and warrant further investigation.
Availability of data and materials
Not applicable.
Abbreviations
AE: Adverse events
ANVISA: National Health Surveillance Agency Brazil
ATP: Adenosine triphosphate
BCAAs: Branched-chain amino acids
BMI: Body mass index
BUN: Blood urea nitrogen
CD14: Cluster of differentiation factor-14
CFU: Colony forming units
CLA: Conjugated linoleic acid
CLR: C-type lectin receptor
CRN: Council for Responsible Nutrition
CRP: C-Reactive protein
EFSA: European Food Safety Authority
FAO: Food and Agricultural Organization
FDA: Food and Drug Administration
FOSHU: Foods for Specific Health Uses
GI: Gastrointestinal
GPR: G-Protein couple receptor
HIV: Human immunodeficiency virus
HPA: Hypothalamic-pituitary-adrenal axis
IBD: Inflammatory bowel disease
ICR: Institute of Cancer Research
IgA: Immunoglobulin A
IL-1β: Interleukin-1beta
IL-6: Interleukin-6
IOC: International Olympic Committee
IPA: International Probiotic Association
ISSN: International Society of Sports Nutrition
LPS: Lipopolysaccharide
MHLW: Ministry of Health and Welfare
NK-κβ: Nuclear factor kappa beta
NOD: Nucleotide-binding oligomerization domain
PAG: Phenylacetylglutamine
PAMP: Pathogen associated molecular pattern
PCR: Polymerase chain reaction
PPR: Pattern recognition receptors
RNA Seq: RNA sequencing
SFCA: Short chain fatty acid
TLR: Toll-like receptor
TMAO: Trimethylamine N-oxide
TNF-α: Tumor necrosis factor-alpha
Treg: Regulatory T cells
URTI: Upper respiratory tract infection
VO2 : Volume of oxygen utilization
WGO: World Gastroenterology Organization
WHO: World Health Organization
References
1.
Hill C, Guarner F, Reid G, Gibson GR, Merenstein DJ, Pot B, Morelli L, Canani RB, Flint HJ, Salminen S, et al. Expert consensus document. The international scientific Association for Probiotics and Prebiotics consensus statement on the scope and appropriate use of the term probiotic. Nat Rev Gastroenterol Hepatol. 2014;11:506–14. https://doi.org/10.1038/nrgastro.2014.66.
Article PubMed PubMed Central Google Scholar
2.
Gibson GR, Hutkins R, Sanders ME, Prescott SL, Reimer RA, Salminen SJ, Scott K, Stanton C, Swanson KS, Cani PD, et al. Expert consensus document: the international scientific Association for Probiotics and Prebiotics (ISAPP) consensus statement on the definition and scope of prebiotics. Nat Rev Gastroenterol Hepatol. 2017;14:491–502. https://doi.org/10.1038/nrgastro.2017.75.
Article PubMed PubMed Central Google Scholar
3.
de Vrese, M.; Schrezenmeir, J. Probiotics, prebiotics, and synbiotics. Adv Biochem Eng Biotechnol 2008, 111, 1–66, doi:https://doi.org/10.1007/10_2008_097.
4.
Aguilar-toala J, Garcia-Perez R, Garcia H, Mata-Haro V, Gonzalez-Cordova A, Vallego-Cordoba B, Hernandez-Mendoza A. Postbiotics: An evolving term within the functional foods field. Trends Food Sci Technol. 2018;75:105–14.
5.
Maughan RJ, Burke LM, Dvorak J, Larson-Meyer DE, Peeling P, Phillips SM, Rawson ES, Walsh NP, Garthe I, Geyer H, et al. IOC consensus statement: dietary supplements and the high-performance athlete. Br J Sports Med. 2018;52:439–55. https://doi.org/10.1136/bjsports-2018-099027.
Article PubMed PubMed Central Google Scholar
6.
Rowland I, Capurso L, Collins K, Cummings J, Delzenne N, Goulet O, Guarner F, Marteau P, Meier R. Current level of consensus on probiotic science–report of an expert meeting–London, 23 November 2009. Gut Microbes. 2010;1:436–9. https://doi.org/10.4161/gmic.1.6.13610.
Article PubMed PubMed Central Google Scholar
7.
Metchnikoff E. The prolongated of life; optimistic studies. New York: G.P. Putnam’s Son; 1908.
8.
Duranti S, Ferrario C, van Sinderen D, Ventura M, Turroni F. Obesity and microbiota: an example of an intricate relationship. Genes Nutr. 2017;12:18. https://doi.org/10.1186/s12263-017-0566-2.
Article PubMed PubMed Central Google Scholar
9.
Staley JT. Biodiversity: are microbial species threatened? Curr Opin Biotechnol. 1997;8:340–5.
PubMed Article CAS PubMed Central Google Scholar
10.
Pyne DB, West NP, Cox AJ, Cripps AW. Probiotics supplementation for athletes – clinical and physiological effects. Eur J Sport Sci. 2015;15:63–72. https://doi.org/10.1080/17461391.2014.971879.
Article PubMed PubMed Central Google Scholar
11.
Costello EK, Lauber CL, Hamady M, Fierer N, Gordon JI, Knight R. Bacterial community variation in human body habitats across space and time. Science. 1694-1697;2009:326. https://doi.org/10.1126/science.1177486.
12.
Duffy LC, Raiten DJ, Hubbard VS, Starke-Reed P. Progress and challenges in developing metabolic footprints from diet in human gut microbial cometabolism. J Nutr. 2015;145:1123S–30S. https://doi.org/10.3945/jn.114.194936.
Article PubMed PubMed Central CAS Google Scholar
13.
Yatsunenko T, Rey FE, Manary MJ, Trehan I, Dominguez-Bello MG, Contreras M, Magris M, Hidalgo G, Baldassano RN, Anokhin AP, et al. Human gut microbiome viewed across age and geography. Nature. 2012;486:222–7. https://doi.org/10.1038/nature11053.
Article PubMed PubMed Central CAS Google Scholar
14.
Zhou Y, Mihindukulasuriya KA, Gao H, La Rosa PS, Wylie KM, Martin JC, Kota K, Shannon WD, Mitreva M, Sodergren E, et al. Exploration of bacterial community classes in major human habitats. Genome Biol. 2014;15:R66. https://doi.org/10.1186/gb-2014-15-5-r66.
Article PubMed PubMed Central Google Scholar
15.
Bressa C, Bailen-Andrino M, Perez-Santiago J, Gonzalez-Soltero R, Perez M, Montalvo-Lominchar MG, Mate-Munoz JL, Dominguez R, Moreno D, Larrosa M. Differences in gut microbiota profile between women with active lifestyle and sedentary women. PLoS One. 2017;12:e0171352. https://doi.org/10.1371/journal.pone.0171352.
Article PubMed PubMed Central CAS Google Scholar
16.
Clarke SF, Murphy EF, O’Sullivan O, Lucey AJ, Humphreys M, Hogan A, Hayes P, O’Reilly M, Jeffery IB, Wood-Martin R, et al. Exercise and associated dietary extremes impact on gut microbial diversity. Gut. 1913-1920;2014:63. https://doi.org/10.1136/gutjnl-2013-306541.
17.
Petersen LM, Bautista EJ, Nguyen H, Hanson BM, Chen L, Lek SH, Sodergren E, Weinstock GM. Community characteristics of the gut microbiomes of competitive cyclists. Microbiome. 2017;5:98. https://doi.org/10.1186/s40168-017-0320-4.
Article PubMed PubMed Central Google Scholar
18.
Morkl S, Lackner S, Muller W, Gorkiewicz G, Kashofer K, Oberascher A, Painold A, Holl A, Holzer P, Meinitzer A, et al. Gut microbiota and body composition in anorexia nervosa inpatients in comparison to athletes, overweight, obese, and normal weight controls. Int J Eat Disord. 2017;50:1421–31. https://doi.org/10.1002/eat.22801.
Article PubMed PubMed Central Google Scholar
19.
Barton W, Penney NC, Cronin O, Garcia-Perez I, Molloy MG, Holmes E, Shanahan F, Cotter PD, O’Sullivan O. The microbiome of professional athletes differs from that of more sedentary subjects in composition and particularly at the functional metabolic level. Gut. 2018;67:625–33. https://doi.org/10.1136/gutjnl-2016-313627.
Article PubMed CAS PubMed Central Google Scholar
20.
Estaki M, Pither J, Baumeister P, Little JP, Gill SK, Ghosh S, Ahmadi-Vand Z, Marsden KR, Gibson DL. Cardiorespiratory fitness as a predictor of intestinal microbial diversity and distinct metagenomic functions. Microbiome. 2016;4:42. https://doi.org/10.1186/s40168-016-0189-7.
Article PubMed PubMed Central Google Scholar
21.
Cerda B, Perez M, Perez-Santiago JD, Tornero-Aguilera JF, Gonzalez-Soltero R, Larrosa M. Gut microbiota modification: another piece in the puzzle of the benefits of physical exercise in health? Front Physiol. 2016;7:51. https://doi.org/10.3389/fphys.2016.00051.
Article PubMed PubMed Central Google Scholar
22.
Choi JJ, Eum SY, Rampersaud E, Daunert S, Abreu MT, Toborek M. Exercise attenuates PCB-induced changes in the mouse gut microbiome. Environ Health Perspect. 2013;121:725–30. https://doi.org/10.1289/ehp.1306534.
Article PubMed PubMed Central Google Scholar
23.
Evans CC, LePard KJ, Kwak JW, Stancukas MC, Laskowski S, Dougherty J, Moulton L, Glawe A, Wang Y, Leone V, et al. Exercise prevents weight gain and alters the gut microbiota in a mouse model of high fat diet-induced obesity. PLoS One. 2014;9:e92193. https://doi.org/10.1371/journal.pone.0092193.
Article PubMed PubMed Central CAS Google Scholar
24.
Petriz BA, Castro AP, Almeida JA, Gomes CP, Fernandes GR, Kruger RH, Pereira RW, Franco OL. Exercise induction of gut microbiota modifications in obese, non-obese and hypertensive rats. BMC Genomics. 2014;15:511. https://doi.org/10.1186/1471-2164-15-511.
Article PubMed PubMed Central Google Scholar
25.
Queipo-Ortuno MI, Seoane LM, Murri M, Pardo M, Gomez-Zumaquero JM, Cardona F, Casanueva F, Tinahones FJ. Gut microbiota composition in male rat models under different nutritional status and physical activity and its association with serum leptin and ghrelin levels. PLoS One. 2013;8:e65465. https://doi.org/10.1371/journal.pone.0065465.
Article PubMed PubMed Central CAS Google Scholar
26.
Campbell SC, Wisniewski PJ, Noji M, McGuinness LR, Haggblom MM, Lightfoot SA, Joseph LB, Kerkhof LJ. The effect of diet and exercise on intestinal integrity and microbial diversity in mice. PLoS One. 2016;11:e0150502. https://doi.org/10.1371/journal.pone.0150502.
Article PubMed PubMed Central CAS Google Scholar
27.
Cook MD, Martin SA, Williams C, Whitlock K, Wallig MA, Pence BD, Woods JA. Forced treadmill exercise training exacerbates inflammation and causes mortality while voluntary wheel training is protective in a mouse model of colitis. Brain Behav Immun. 2013;33:46–56. https://doi.org/10.1016/j.bbi.2013.05.005.
Article PubMed PubMed Central Google Scholar
28.
Allen JM, Mailing LJ, Niemiro GM, Moore R, Cook MD, White BA, Holscher HD, Woods JA. Exercise alters gut microbiota composition and function in lean and obese humans. Med Sci Sports Exerc. 2018;50:747–57. https://doi.org/10.1249/MSS.0000000000001495.
Article PubMed PubMed Central Google Scholar
29.
Matsumoto M, Inoue R, Tsukahara T, Ushida K, Chiji H, Matsubara N, Hara H. Voluntary running exercise alters microbiota composition and increases n-butyrate concentration in the rat cecum. Biosci Biotechnol Biochem. 2008;72:572–6. https://doi.org/10.1271/bbb.70474.
Article PubMed CAS PubMed Central Google Scholar
30.
Scheiman J, Luber JM, Chavkin TA, MacDonald T, Tung A, Pham LD, Wibowo MC, Wurth RC, Punthambaker S, Tierney BT, et al. Meta-omics analysis of elite athletes identifies a performance-enhancing microbe that functions via lactate metabolism. Nat Med. 2019;25:1104–9. https://doi.org/10.1038/s41591-019-0485-4.
Article PubMed CAS PubMed Central Google Scholar
31.
Cronin O, O’Sullivan O, Barton W, Cotter PD, Molloy MG, Shanahan F. Gut microbiota: implications for sports and exercise medicine. Br J Sports Med. 2017;51:700–1. https://doi.org/10.1136/bjsports-2016-097225.
Article PubMed PubMed Central Google Scholar
32.
David LA, Maurice CF, Carmody RN, Gootenberg DB, Button JE, Wolfe BE, Ling AV, Devlin AS, Varma Y, Fischbach MA, et al. Diet rapidly and reproducibly alters the human gut microbiome. Nature. 2014;505:559–63. https://doi.org/10.1038/nature12820.
Article PubMed CAS PubMed Central Google Scholar
33.
Wu GD, Chen J, Hoffmann C, Bittinger K, Chen YY, Keilbaugh SA, Bewtra M, Knights D, Walters WA, Knight R, et al. Linking long-term dietary patterns with gut microbial enterotypes. Science. 2011;334:105–8. https://doi.org/10.1126/science.1208344.
Article PubMed PubMed Central CAS Google Scholar
34.
Sheflin AM, Melby CL, Carbonero F, Weir TL. Linking dietary patterns with gut microbial composition and function. Gut Microbes. 2017;8:113–29. https://doi.org/10.1080/19490976.2016.1270809.
Article PubMed CAS PubMed Central Google Scholar
35.
Moreno-Perez D, Bressa C, Bailen M, Hamed-Bousdar S, Naclerio F, Carmona M, Perez M, Gonzalez-Soltero R, Montalvo-Lominchar MG, Carabana C, et al. Effect of a protein supplement on the gut microbiota of endurance athletes: a randomized, controlled, Double-Blind Pilot Study. Nutrients. 2018;10. https://doi.org/10.3390/nu10030337.
PubMed Central Article CAS Google Scholar
36.
Gleeson M. Immune function in sport and exercise. J App Physiol (1985). 2007;103:693–9. https://doi.org/10.1152/japplphysiol.00008.2007.
37.
Tap J, Furet JP, Bensaada M, Philippe C, Roth H, Rabot S, Lakhdari O, Lombard V, Henrissat B, Corthier G, et al. Gut microbiota richness promotes its stability upon increased dietary fibre intake in healthy adults. Environ Microbiol. 2015;17:4954–64. https://doi.org/10.1111/1462-2920.13006.
Article PubMed CAS PubMed Central Google Scholar
38.
Cramp T, Broad E, Martin D, Meyer BJ. Effects of preexercise carbohydrate ingestion on mountain bike performance. Med Sci Sports Exerc. 1602-1609;2004:36.
39.
Caesar R, Tremaroli V, Kovatcheva-Datchary P, Cani PD, Backhed F. Crosstalk between gut microbiota and dietary lipids aggravates WAT inflammation through TLR signaling. Cell Metab. 2015;22:658–68. https://doi.org/10.1016/j.cmet.2015.07.026.
Article PubMed PubMed Central CAS Google Scholar
40.
Rawson ES, Miles MP, Larson-Meyer DE. Dietary supplements for health, adaptation, and recovery in athletes. Int J Sport Nutr Exerc Metab. 2018;28:188–99. https://doi.org/10.1123/ijsnem.2017-0340.
Article PubMed CAS PubMed Central Google Scholar
41.
de Oliveira EP, Burini RC, Jeukendrup A. Gastrointestinal complaints during exercise: prevalence, etiology, and nutritional recommendations. Sports Med. 2014;44(Suppl 1):S79–85. https://doi.org/10.1007/s40279-014-0153-2.
Article PubMed PubMed Central Google Scholar
42.
Jäger R, Purpura M, Stone JD, Turner SM, Anzalone AJ, Eimerbrink MJ, Pane M, Amoruso A, Rowlands DS, Oliver JM. Probiotic Streptococcus thermophilus FP4 and Bifidobacterium breve BR03 supplementation attenuates performance and range-of-motion decrements following muscle damaging exercise. Nutrients. 2016;8. https://doi.org/10.3390/nu8100642.
PubMed Central Article CAS Google Scholar
43.
Jäger R, Shields KA, Lowery RP, De Souza EO, Partl JM, Hollmer C, Purpura M, Wilson JM. Probiotic Bacillus coagulans GBI-30, 6086 reduces exercise-induced muscle damage and increases recovery. PeerJ. 2016;4:e2276. https://doi.org/10.7717/peerj.2276.
PubMed PubMed Central Article CAS Google Scholar
44.
Salarkia N, Ghadamli L, Zaeri F, Sabaghian Rad L. Effects of probiotic yogurt on performance, respiratory and digestive systems of young adult female endurance swimmers: a randomized controlled trial. Med J Islam Repub Iran. 2013;27:141–6.
PubMed PubMed Central Google Scholar
45.
Salehzadeh K. The effects of probiotic yogurt drink on lipid profile, CRP, and record changes in aerobic athletes. Life Sci. 2015;9:32–7.
46.
Shing CM, Peake JM, Lim CL, Briskey D, Walsh NP, Fortes MB, Ahuja KD, Vitetta L. Effects of probiotics supplementation on gastrointestinal permeability, inflammation and exercise performance in the heat. Eur J Appl Physiol. 2014;114:93–103. https://doi.org/10.1007/s00421-013-2748-y.
Article PubMed PubMed Central Google Scholar
47.
Klaenhammer TR, Kleerebezem M, Kopp MV, Rescigno M. The impact of probiotics and prebiotics on the immune system. Nat Rev Immunol. 2012;12:728–34. https://doi.org/10.1038/nri3312.
Article PubMed CAS PubMed Central Google Scholar
48.
Sang LX, Chang B, Zhang WL, Wu XM, Li XH, Jiang M. Remission induction and maintenance effect of probiotics on ulcerative colitis: a meta-analysis. World J Gastroenterol. 1908-1915;2010:16.
49.
Ng SC, Hart AL, Kamm MA, Stagg AJ, Knight SC. Mechanisms of action of probiotics: recent advances. Inflamm Bowel Dis. 2009;15:300–10. https://doi.org/10.1002/ibd.20602.
Article PubMed CAS PubMed Central Google Scholar
50.
Plaza-Diaz J, Gomez-Llorente C, Fontana L, Gil A. Modulation of immunity and inflammatory gene expression in the gut, in inflammatory diseases of the gut and in the liver by probiotics. World J Gastroenterol. 2014;20:15632–49. https://doi.org/10.3748/wjg.v20.i42.15632.
Article PubMed PubMed Central CAS Google Scholar
51.
Miettinen M, Vuopio-Varkila J, Varkila K. Production of human tumor necrosis factor alpha, interleukin-6, and interleukin-10 is induced by lactic acid bacteria. Infect Immun. 1996;64:5403–5.
PubMed PubMed Central CAS Google Scholar
52.
Nazemian V, Shadnoush M, Manaheji H, Zaringhalam J. Probiotics and inflammatory pain: a literature review study. Middle East J Rehab Health. 2016;3:e36087.
53.
Clancy RL, Gleeson M, Cox A, Callister R, Dorrington M, D’Este C, Pang G, Pyne D, Fricker P, Henriksson A. Reversal in fatigued athletes of a defect in interferon gamma secretion after administration of Lactobacillus acidophilus. Br J Sports Med. 2006;40:351–4. https://doi.org/10.1136/bjsm.2005.024364.
Article PubMed PubMed Central CAS Google Scholar
54.
Moreira A, Kekkonen R, Korpela R, Delgado L, Haahtela T. Allergy in marathon runners and effect of Lactobacillus GG supplementation on allergic inflammatory markers. Respir Med. 2007;101:1123–31. https://doi.org/10.1016/j.rmed.2006.11.015.
Article PubMed PubMed Central Google Scholar
55.
Kekkonen RA, Vasankari TJ, Vuorimaa T, Haahtela T, Julkunen I, Korpela R. The effect of probiotics on respiratory infections and gastrointestinal symptoms during training in marathon runners. Int J Sport Nutr Exerc Metab. 2007;17:352–63.
PubMed Article PubMed Central Google Scholar
56.
Tiollier E, Chennaoui M, Gomez-Merino D, Drogou C, Filaire E, Guezennec CY. Effect of a probiotics supplementation on respiratory infections and immune and hormonal parameters during intense military training. Mil Med. 2007;172:1006–11. https://doi.org/10.7205/milmed.172.9.1006.
Article PubMed PubMed Central Google Scholar
57.
Cox AJ, Pyne DB, Saunders PU, Fricker PA. Oral administration of the probiotic Lactobacillus fermentum VRI-003 and mucosal immunity in endurance athletes. Br J Sports Med. 2010;44:222–6. https://doi.org/10.1136/bjsm.2007.044628.
Article PubMed CAS PubMed Central Google Scholar
58.
Martarelli D, Verdenelli MC, Scuri S, Cocchioni M, Silvi S, Cecchini C, Pompei P. Effect of a probiotic intake on oxidant and antioxidant parameters in plasma of athletes during intense exercise training. Curr Microbiol. 1689-1696;2011:62. https://doi.org/10.1007/s00284-011-9915-3.
59.
Gleeson M, Bishop NC, Stensel DJ, Lindley MR, Mastana SS, Nimmo MA. The anti-inflammatory effects of exercise: mechanisms and implications for the prevention and treatment of disease. Nat Rev Immunol. 2011;11:607–15. https://doi.org/10.1038/nri3041.
Article PubMed CAS PubMed Central Google Scholar
60.
Gleeson M, Bishop NC, Oliveira M, Tauler P. Daily probiotic’s (Lactobacillus casei Shirota) reduction of infection incidence in athletes. Int J Sport Nutr Exerc Metab. 2011;21:55–64.
PubMed Article PubMed Central Google Scholar
61.
West NP, Pyne DB, Cripps AW, Hopkins WG, Eskesen DC, Jairath A, Christophersen CT, Conlon MA, Fricker PA. Lactobacillus fermentum (PCC(R)) supplementation and gastrointestinal and respiratory-tract illness symptoms: a randomised control trial in athletes. Nutr J. 2011;10:30. https://doi.org/10.1186/1475-2891-10-30.
Article PubMed PubMed Central Google Scholar
62.
Välimäki et al. Int J Sports Med. 2012;33(4):291–6. https://doi.org/10.1055/s-0031-1291223.
PubMed Article CAS PubMed Central Google Scholar
63.
Lamprecht M, Bogner S, Schippinger G, Steinbauer K, Fankhauser F, Hallstroem S, Schuetz B, Greilberger JF. Probiotic supplementation affects markers of intestinal barrier, oxidation, and inflammation in trained men; a randomized, double-blinded, placebo-controlled trial. J Int Soc Sports Nutr. 2012;9:45. https://doi.org/10.1186/1550-2783-9-45.
Article PubMed PubMed Central CAS Google Scholar
64.
Gleeson M, Bishop N, Oliveira M, McCauley T, Tauler P, Muhamad AS. Respiratory infection risk in athletes: association with antigen-stimulated IL-10 production and salivary IgA secretion. Scand J Med Sci Sports. 2012;22:410–7. https://doi.org/10.1111/j.1600-0838.2010.01272.x.
Article PubMed CAS PubMed Central Google Scholar
65.
Grobbelaar et al. The influence of probiotic supplementation on selected athletic performance-related blood markers in men. African Journal for Physical, Health Education, Recreation and Dance. 2012;18(1):1–11.
66.
West NP, Pyne DB, Cripps AW, Christophersen CT, Conlon MA, Fricker PA. Gut balance, a synbiotic supplement, increases fecal Lactobacillus paracasei but has little effect on immunity in healthy physically active individuals. Gut Microbes. 2012;3:221–7. https://doi.org/10.4161/gmic.19579.
Article PubMed PubMed Central Google Scholar
67.
Sashihara T, Nagata M, Mori T, Ikegami S, Gotoh M, Okubo K, Uchida M, Itoh H. Effects of Lactobacillus gasseri OLL2809 and alpha-lactalbumin on university-student athletes: a randomized, double-blind, placebo-controlled clinical trial. Appl Physiol Nutr Metab. 2013;38:1228–35. https://doi.org/10.1139/apnm-2012-0490.
Article PubMed CAS PubMed Central Google Scholar
68.
West NP, Horn PL, Pyne DB, Gebski VJ, Lahtinen SJ, Fricker PA, Cripps AW. Probiotic supplementation for respiratory and gastrointestinal illness symptoms in healthy physically active individuals. Clin Nutr. 2014;33:581–7. https://doi.org/10.1016/j.clnu.2013.10.002.
Article PubMed CAS PubMed Central Google Scholar
69.
Haywood BA, Black KE, Baker D, McGarvey J, Healey P, Brown RC. Probiotic supplementation reduces the duration and incidence of infections but not severity in elite rugby union players. J Sci Med Sport. 2014;17:356–60. https://doi.org/10.1016/j.jsams.2013.08.004.
Article PubMed PubMed Central Google Scholar
70.
Aghaee M, Khosravi N, Hanachi P, Kordi MR, Aghaee R. Effect of probiotic supplement on immune response in male athletes. Qom Univ Med Sci. 2014;7:27–33.
71.
Georges J, Lowery R, Yaman G, Kerio C, Ormes J, McCleary S, Sharp M, Shields K, Rauch J, Silva J, et al. The effects of probiotic supplementation on lean body mass, strength, power, and health indicators in resistance trained males: a pilot study. J Int Soc Sports Nutr. 2014;11:P38.
PubMed Central Article Google Scholar
72.
Narimani-Rad et al. Indian Journal of Fundamental and Applied Life Sciences (Online) An Open Access, Online International Journal Available at www.cibtech.org/sp.ed/jls/2014/03/jls.htm. 2014;4(S3):231–5. ISSN: 2231–6345.
73.
Muhamad A, Gleeson M. Effects of a 14-strain probiotics supplement on salivary antimicrobial proteins at rest and in reponse to an acute bout of prolonged exercise. Int J Sports Sci. 2014;4:60–6.
74.
O’Brien KV, Stewart LK, Forney LA, Aryana KJ, Prinyawiwatkul W, Boeneke CA. The effects of postexercise consumption of a kefir beverage on performance and recovery during intensive endurance training. J Dairy Sci. 2015;98:7446–9. https://doi.org/10.3168/jds.2015-9392.
Article PubMed CAS PubMed Central Google Scholar
75.
Gill SK, Teixeira AM, Rosado F, Cox M, Costa RJ. High-dose probiotic supplementation containing Lactobacillus casei for 7 days does not enhance salivary antimicrobial protein responses to exertional heat stress compared with placebo. Int J Sport Nutr Exerc Metab. 2016;26:150–60. https://doi.org/10.1123/ijsnem.2015-0171.
Article PubMed CAS PubMed Central Google Scholar
76.
Gill SK, Allerton DM, Ansley-Robson P, Hemmings K, Cox M, Costa RJ. Does short-term high dose probiotic supplementation containing Lactobacillus casei attenuate exertional-heat stress induced Endotoxaemia and Cytokinaemia? Int J Sport Nutr Exerc Metab. 2016;26:268–75. https://doi.org/10.1123/ijsnem.2015-0186.
Article PubMed CAS PubMed Central Google Scholar
77.
Roberts JD, Suckling CA, Peedle GY, Murphy JA, Dawkins TG, Roberts MG. An exploratory investigation of endotoxin levels in novice long distance triathletes, and the effects of a multi-strain probiotic/prebiotic. Antioxidant Intervention Nutrients. 2016;8. https://doi.org/10.3390/nu8110733.
PubMed Central Article Google Scholar
78.
Strasser B, Geiger D, Schauer M, Gostner JM, Gatterer H, Burtscher M, Fuchs D. Probiotic supplements beneficially affect tryptophan-kynurenine metabolism and reduce the incidence of upper respiratory tract infections in trained athletes: a randomized, double-blinded, Placebo-Controlled Trial. Nutrients. 2016;8. https://doi.org/10.3390/nu8110752.
PubMed Central Article Google Scholar
79.
Michalickova D, Minic R, Dikic N, Andjelkovic M, Kostic-Vucicevic M, Stojmenovic T, Nikolic I, Djordjevic B. Lactobacillus helveticus Lafti L10 supplementation reduces respiratory infection duration in a cohort of elite athletes: a randomized, double-blind, placebo-controlled trial. Appl Physiol Nutr Metab. 2016;41:782–9. https://doi.org/10.1139/apnm-2015-0541.
Article PubMed CAS PubMed Central Google Scholar
80.
Gleeson M, Bishop NC, Struszczak L. Effects of Lactobacillus casei Shirota ingestion on common cold infection and herpes virus antibodies in endurance athletes: a placebo-controlled, randomized trial. Eur J Appl Physiol. 2016;116:1555–63. https://doi.org/10.1007/s00421-016-3415-x.
Article PubMed PubMed Central CAS Google Scholar
81.
Marshall H, Chrismas BCR, Suckling CA, Roberts JD, Foster J, Taylor L. Chronic probiotic supplementation with or without glutamine does not influence the eHsp72 response to a multi-day ultra-endurance exercise event. Appl Physiol Nutr Metab. 2017;42:876–83. https://doi.org/10.1139/apnm-2017-0131.
Article PubMed CAS PubMed Central Google Scholar
82.
Brennan et al. Med. Sci. Sports Exerc. 2018;50:840. https://doi.org/10.1249/01.mss.0000538764.55259.9c.
83.
Townsend JR, Bender D, Vantrease WC, Sapp PA, Toy AM, Woods CA, Johnson KD. Effects of Probiotic (Bacillus subtilis DE111) Supplementation on Immune Function, Hormonal Status, and Physical Performance in Division I Baseball Players. Sports (Basel). 2018:6. https://doi.org/10.3390/sports6030070.
PubMed Central Article Google Scholar
84.
Antonio J, Leaf A, Carson C, Ellerbroek A, Axelrod C, Silver T, Burgess V, Peacock C. The effects of probiotic supplementation in active men and women. J Exerc Nutr. 2018;1.
85.
Huang WC, Hsu YJ, Li H, Kan NW, Chen YM, Lin JS, Hsu TK, Tsai TY, Chiu YS, Huang CC. Effect of Lactobacillus Plantarum TWK10 on improving endurance performance in humans. Chin J Phys. 2018;61:163–70. https://doi.org/10.4077/CJP.2018.BAH587.
86.
Carbuhn AF, Reynolds SM, Campbell CW, Bradford LA, Deckert JA, Kreutzer A. Fry, A.C. Effects of Probiotic (Bifidobacterium longum 35624) Supplementation on Exercise Performance, Immune Modulation, and Cognitive Outlook in Division I Female Swimmers. Sports (Basel). 2018:6. https://doi.org/10.3390/sports6040116.
PubMed Central Article Google Scholar
87.
Huang et al. Nutrients. 2019;11(2). https://doi.org/10.3390/nu11020353.
PubMed Central Article CAS Google Scholar
88.
Pugh et al. Eur J Appl Physiol. 2019;119(7):1491–501. https://doi.org/10.1007/s00421-019-04136-3.
PubMed PubMed Central Article Google Scholar
89.
Pumpa et al. J Sci Med Sport. 2019;22(8):876–81. https://doi.org/10.1016/j.jsams.2019.03.013.
PubMed Article PubMed Central Google Scholar
90.
Vaisberg M, Paixao V, Almeida EB, Santos JMB, Foster R, Rossi M, Pithon-Curi TC, Gorjao R, Momesso CM, Andrade MS, et al. Daily intake of fermented Milk containing Lactobacillus casei Shirota (Lcs) modulates systemic and upper airways immune/inflammatory responses in Marathon runners. Nutrients. 2019;11. https://doi.org/10.3390/nu11071678.
PubMed Central Article Google Scholar
91.
Meeusen R. Exercise, nutrition and the brain. Sports Med. 2014;44(Suppl 1):S47–56. https://doi.org/10.1007/s40279-014-0150-5.
Article PubMed PubMed Central Google Scholar
92.
Chen YM, Wei L, Chiu YS, Hsu YJ, Tsai TY, Wang MF, Huang CC. Lactobacillus plantarum TWK10 supplementation improves exercise performance and increases muscle mass in mice. Nutrients. 2016;8:205. https://doi.org/10.3390/nu8040205.
Article PubMed PubMed Central CAS Google Scholar
93.
Wang X, Shao C, Liu L, Guo X, Xu Y, Lu X. Optimization, partial characterization and antioxidant activity of an exopolysaccharide from Lactobacillus plantarum KX041. Int J Biol Macromol. 2017;103:1173–84. https://doi.org/10.1016/j.ijbiomac.2017.05.118.
Article PubMed CAS PubMed Central Google Scholar
94.
Storelli G, Defaye A, Erkosar B, Hols P, Royet J, Leulier F. Lactobacillus plantarum promotes Drosophila systemic growth by modulating hormonal signals through TOR-dependent nutrient sensing. Cell Metab. 2011;14:403–14. https://doi.org/10.1016/j.cmet.2011.07.012.
Article PubMed CAS PubMed Central Google Scholar
95.
Schwarzer M, Makki K, Storelli G, Machuca-Gayet I, Srutkova D, Hermanova P, Martino ME, Balmand S, Hudcovic T, Heddi A, et al. Lactobacillus plantarum strain maintains growth of infant mice during chronic undernutrition. Science. 2016;351:854–7. https://doi.org/10.1126/science.aad8588.
Article PubMed CAS PubMed Central Google Scholar
96.
Minevich J, Olson M, Mannion J, Boublik J, McPerson J, Lowery R, Shields K, Sharp M, De Souza E, Wilson J, et al. Digestive enzymes reduce quality difference between plant and animal proteins: a double-blind crossover study (abstract). J Int Soc Sports Nutr. 2015;12:P26.
PubMed Central Article Google Scholar
97.
Wang Y, Gu Q. Effect of probiotic on growth performance and digestive enzyme activity of arbor acres broilers. Res Vet Sci. 2010;89:163–7. https://doi.org/10.1016/j.rvsc.2010.03.009.
Article PubMed CAS PubMed Central Google Scholar
98.
Kimmel M, Keller D, Farmer S, Warrino DE. A controlled clinical trial to evaluate the effect of GanedenBC(30) on immunological markers. Methods Find Exp Clin Pharmacol. 2010;32:129–32. https://doi.org/10.1358/mf.2010.32.2.1423881.
Article PubMed CAS PubMed Central Google Scholar
99.
Maathuis AJ, Keller D, Farmer S. Survival and metabolic activity of the GanedenBC30 strain of Bacillus coagulans in a dynamic in vitro model of the stomach and small intestine. Benefic Microbes. 2010;1:31–6. https://doi.org/10.3920/BM2009.0009.
100.
Klemenak M, Dolinsek J, Langerholc T, Di Gioia D, Micetic-Turk D. Administration of Bifidobacterium breve decreases the production of TNF-alpha in children with celiac disease. Dig Dis Sci. 2015;60:3386–92. https://doi.org/10.1007/s10620-015-3769-7.
Article PubMed CAS PubMed Central Google Scholar
101.
Nicola S, Mogna L, Allesina S, Barba M, Deidda F, Lorenzini P, Raiteri E, Strozzi G, Mogna G. Interaction between probiotics and human immune cells. The prospective anti-inflammatory activity of Bifidobacterium breve BRO3. Agro Food Ind Hi-Tech. 2010;21:9–13.
102.
Saggioro A. Probiotics in the treatment of irritable bowel syndrome. J Clin Gastroenterol. 2004;38:S104–6.
PubMed Article PubMed Central Google Scholar
103.
Toohey JC, Townsend JR, Johnson SB, Toy AM, Vantrease WC, Bender D, Crimi CC, Stowers KL, Ruiz MD, VanDusseldorp TA, et al. Effects of probiotic (Bacillus subtilis) supplementation during offseason resistance training in female division I athletes. J Strength Cond Res. 2018. https://doi.org/10.1519/JSC.0000000000002675.
104.
Mekkes MC, Weenen TC, Brummer RJ, Claassen E. The development of probiotic treatment in obesity: a review. Benefic Microbes. 2014;5:19–28. https://doi.org/10.3920/BM2012.0069.
105.
Minami J, Kondo S, Yanagisawa N, Odamaki T, Xiao JZ, Abe F, Nakajima S, Hamamoto Y, Saitoh S, Shimoda T. Oral administration of Bifidobacterium breve B-3 modifies metabolic functions in adults with obese tendencies in a randomised controlled trial. J Nutr Sci. 2015;4:e17. https://doi.org/10.1017/jns.2015.5.
Article PubMed PubMed Central CAS Google Scholar
106.
Kondo S, Xiao JZ, Satoh T, Odamaki T, Takahashi S, Sugahara H, Yaeshima T, Iwatsuki K, Kamei A, Abe K. Antiobesity effects of Bifidobacterium breve strain B-3 supplementation in a mouse model with high-fat diet-induced obesity. Biosci Biotechnol Biochem. 1656-1661;2010:74. https://doi.org/10.1271/bbb.100267.
107.
Hardin BJ, Campbell KS, Smith JD, Arbogast S, Smith J, Moylan JS, Reid MB. TNF-alpha acts via TNFR1 and muscle-derived oxidants to depress myofibrillar force in murine skeletal muscle. J App Physiol (1985). 2008;104:694–9. https://doi.org/10.1152/japplphysiol.00898.2007.
108.
Lang CH, Frost RA, Nairn AC, MacLean DA, Vary TC. TNF-alpha impairs heart and skeletal muscle protein synthesis by altering translation initiation. Am J Physiol Endocrinol Metab. 2002;282:E336–47. https://doi.org/10.1152/ajpendo.00366.2001.
Article PubMed CAS PubMed Central Google Scholar
109.
Main LC, Dawson B, Heel K, Grove JR, Landers GJ, Goodman C. Relationship between inflammatory cytokines and self-report measures of training overload. Res Sports Med (Print). 2010;18:127–39. https://doi.org/10.1080/15438621003627133.
110.
Kristensen NB, Bryrup T, Allin KH, Nielsen T, Hansen TH, Pedersen O. Alterations in fecal microbiota composition by probiotic supplementation in healthy adults: a systematic review of randomized controlled trials. Genome Med. 2016;8:52. https://doi.org/10.1186/s13073-016-0300-5.
Article PubMed PubMed Central CAS Google Scholar
111.
McFarland LV. Use of probiotics to correct dysbiosis of normal microbiota following disease or disruptive events: a systematic review. BMJ Open. 2014;4:e005047. https://doi.org/10.1136/bmjopen-2014-005047.
Article PubMed PubMed Central Google Scholar
112.
Turnbaugh PJ, Hamady M, Yatsunenko T, Cantarel BL, Duncan A, Ley RE, Sogin ML, Jones WJ, Roe BA, Affourtit JP, et al. A core gut microbiome in obese and lean twins. Nature. 2009;457:480–4. https://doi.org/10.1038/nature07540.
Article PubMed CAS PubMed Central Google Scholar
113.
Donmez N, Kisadere I, Balaban C, Kadiralieva N. Effects of traditional homemade koumiss on some hematological and biochemical characteristics in sedentary men exposed to exercise. Biotech Histochem. 2014;89:558–63. https://doi.org/10.3109/10520295.2014.915428.
Article PubMed CAS PubMed Central Google Scholar
114.
Lollo PC, Cruz AG, Morato PN, Moura CS, Carvalho-Silva LB, Oliveira CA, Faria JA, Amaya-Farfan J. Probiotic cheese attenuates exercise-induced immune suppression in Wistar rats. J Dairy Sci. 2012;95:3549–58. https://doi.org/10.3168/jds.2011-5124.
Article PubMed CAS PubMed Central Google Scholar
115.
Wells JM, Rossi O, Meijerink M, van Baarlen P. Epithelial crosstalk at the microbiota-mucosal interface. Proc Natl Acad Sci U S A. 2011;108(Suppl 1):4607–14. https://doi.org/10.1073/pnas.1000092107.
Article PubMed PubMed Central Google Scholar
116.
Schwellnus M, Soligard T, Alonso JM, Bahr R, Clarsen B, Dijkstra HP, Gabbett TJ, Gleeson M, Hagglund M, Hutchinson MR, et al. How much is too much? (part 2) International Olympic Committee consensus statement on load in sport and risk of illness. Br J Sports Med. 2016;50:1043–52. https://doi.org/10.1136/bjsports-2016-096572.
Article PubMed PubMed Central Google Scholar
117.
Geuking MB, McCoy KD, Macpherson AJ. Metabolites from intestinal microbes shape Treg. Cell Res. 2013;23:1339–40. https://doi.org/10.1038/cr.2013.125.
Article PubMed PubMed Central CAS Google Scholar
118.
Hiramatsu Y, Hosono A, Konno T, Nakanishi Y, Muto M, Suyama A, Hachimura S, Sato R, Takahashi K, Kaminogawa S. Orally administered Bifidobacterium triggers immune responses following capture by CD11c(+) cells in Peyer’s patches and cecal patches. Cytotechnology. 2011;63:307–17. https://doi.org/10.1007/s10616-011-9349-6.
Article PubMed PubMed Central Google Scholar
119.
Shephard RJ. Special feature for the Olympics: effects of exercise on the immune system: overview of the epidemiology of exercise immunology. Immunol Cell Biol. 2000;78:485–95. https://doi.org/10.1111/j.1440-1711.2000.t01-1-.x.
Article PubMed CAS PubMed Central Google Scholar
120.
Chakravarti A. The CD4/CD8 ratio: message in a bottle? Nat Med. 1995;1:1240–1. https://doi.org/10.1038/nm1295-1240.
Article PubMed CAS PubMed Central Google Scholar
121.
Sanders M, Merenstein D, Merrifield C, Hutkins R. Probiotics for Human Use. Nutr Bull. 2019;43:212–25.
122.
Azad MAK, Sarker M, Wan D. Immunomodulatory effects of probiotics on cytokine profiles. Biomed Res Int. 2018;2018:8063647. https://doi.org/10.1155/2018/8063647.
Article PubMed PubMed Central CAS Google Scholar
123.
Šušković J, Kos B, Novak J, Pavunc A, Habjanič K, Matoć S. Antimicrobial activity – the most important property of probiotic and starter lactic acid bacteria. Food Technol Biotechnol. 2010;48:296–307.
124.
Dobson A, Cotter PD, Ross RP, Hill C. Bacteriocin production: a probiotic trait? Appl Environ Microbiol. 2012;78:1–6. https://doi.org/10.1128/AEM.05576-11.
Article PubMed PubMed Central CAS Google Scholar
125.
Cotter PD, Hill C, Ross RP. Bacteriocins: developing innate immunity for food. Nat Rev Microbiol. 2005;3:777–88. https://doi.org/10.1038/nrmicro1273.
Article PubMed CAS PubMed Central Google Scholar
126.
Wosinska L, Cotter PD, O’Sullivan O, Guinane C. The potential impact of probiotics on the gut microbiome of athletes. Nutrients. 2019;11. https://doi.org/10.3390/nu11102270.
PubMed Central Article Google Scholar
127.
Rehrer NJ, van Kemenade M, Meester W, Brouns F, Saris WH. Gastrointestinal complaints in relation to dietary intake in triathletes. Int J Sport Nutr. 1992;2:48–59.
PubMed Article CAS PubMed Central Google Scholar
128.
van Wijck K, Lenaerts K, Grootjans J, Wijnands KA, Poeze M, van Loon LJ, Dejong CH, Buurman WA. Physiology and pathophysiology of splanchnic hypoperfusion and intestinal injury during exercise: strategies for evaluation and prevention. Am J Physiol Gastrointest Liver Physiol. 2012;303:G155–68. https://doi.org/10.1152/ajpgi.00066.2012.
Article PubMed CAS PubMed Central Google Scholar
129.
van Wijck K, Lenaerts K, van Loon LJ, Peters WH, Buurman WA, Dejong CH. Exercise-induced splanchnic hypoperfusion results in gut dysfunction in healthy men. PLoS One. 2011;6:e22366. https://doi.org/10.1371/journal.pone.0022366.
Article PubMed PubMed Central CAS Google Scholar
130.
Jeukendrup AE, Jentjens RL, Moseley L. Nutritional considerations in triathlon. Sports Med. 2005;35:163–81 doi:3525 [pii].
PubMed Article PubMed Central Google Scholar
131.
Jeukendrup AE. Training the gut for athletes. Sports Med. 2017;47:101–10. https://doi.org/10.1007/s40279-017-0690-6.
Article PubMed PubMed Central Google Scholar
132.
Brennan, C.J.; Axelrod, C.; Paul, D.; Hull, M.; Kirwan, J.P. Effects of a novel probiotic on exercise-induced gut permeability and microbiota in endurance athletes (abstract). In proceedings of American College of Sports Medicine, Minneapolis, MN.
133.
Fasano A. Zonulin and its regulation of intestinal barrier function: the biological door to inflammation, autoimmunity, and cancer. Physiol Rev. 2011;91:151–75. https://doi.org/10.1152/physrev.00003.2008.
Article PubMed CAS PubMed Central Google Scholar
134.
de Oliveira EP, Burini RC. Food-dependent, exercise-induced gastrointestinal distress. J Int Soc Sports Nutr. 2011;8:12. https://doi.org/10.1186/1550-2783-8-12.
Article PubMed PubMed Central CAS Google Scholar
135.
Groschwitz KR, Hogan SP. Intestinal barrier function: molecular regulation and disease pathogenesis. J Allergy Clin Immunol. 2009;124:3–20; quiz 21-22. https://doi.org/10.1016/j.jaci.2009.05.038.
Article PubMed PubMed Central CAS Google Scholar
136.
Sonier B, Patrick C, Ajjikuttira P, Scott FW. Intestinal immune regulation as a potential diet-modifiable feature of gut inflammation and autoimmunity. Int Rev Immunol. 2009;28:414–45. https://doi.org/10.3109/08830180903208329.
Article PubMed CAS PubMed Central Google Scholar
137.
Jeukendrup AE, Vet-Joop K, Sturk A, Stegen JH, Senden J, Saris WH, Wagenmakers AJ. Relationship between gastro-intestinal complaints and endotoxaemia, cytokine release and the acute-phase reaction during and after a long-distance triathlon in highly trained men. Clin Sci (Lond). 2000;98:47–55.
138.
Reid G. Probiotics: definition, scope and mechanisms of action. Best Pract Res Clin Gastroenterol. 2016;30:17–25. https://doi.org/10.1016/j.bpg.2015.12.001.
Article PubMed CAS PubMed Central Google Scholar
139.
Langa S, Martin-Cabrejas I, Montiel R, Landete JM, Medina M, Arques JL. Short communication: combined antimicrobial activity of reuterin and diacetyl against foodborne pathogens. J Dairy Sci. 2014;97:6116–21. https://doi.org/10.3168/jds.2014-8306.
Article PubMed CAS PubMed Central Google Scholar
140.
Velraeds MM, van de Belt-Gritter B, van der Mei HC, Reid G, Busscher HJ. Interference in initial adhesion of uropathogenic bacteria and yeasts to silicone rubber by a Lactobacillus acidophilus biosurfactant. J Med Microbiol. 1998;47:1081–5. https://doi.org/10.1099/00222615-47-12-1081.
Article PubMed CAS PubMed Central Google Scholar
141.
Bermudez-Brito M, Plaza-Diaz J, Munoz-Quezada S, Gomez-Llorente C, Gil A. Probiotic mechanisms of action. Ann Nutr Metab. 2012;61:160–74. https://doi.org/10.1159/000342079.
Article PubMed CAS PubMed Central Google Scholar
142.
Ohland CL, Macnaughton WK. Probiotic bacteria and intestinal epithelial barrier function. Am J Physiol Gastrointest Liver Physiol. 2010;298:G807–19. https://doi.org/10.1152/ajpgi.00243.2009.
Article PubMed CAS PubMed Central Google Scholar
143.
Hooper LV, Stappenbeck TS, Hong CV, Gordon JI. Angiogenins: a new class of microbicidal proteins involved in innate immunity. Nat Immunol. 2003;4:269–73. https://doi.org/10.1038/ni888.
Article PubMed CAS PubMed Central Google Scholar
144.
Hooper LV, Wong MH, Thelin A, Hansson L, Falk PG, Gordon JI. Molecular analysis of commensal host-microbial relationships in the intestine. Science. 2001;291:881–4. https://doi.org/10.1126/science.291.5505.881.
Article PubMed CAS PubMed Central Google Scholar
145.
Anderson RC, Cookson AL, McNabb WC, Park Z, McCann MJ, Kelly WJ, Roy NC. Lactobacillus plantarum MB452 enhances the function of the intestinal barrier by increasing the expression levels of genes involved in tight junction formation. BMC Microbiol. 2010;10:316. https://doi.org/10.1186/1471-2180-10-316.
Article PubMed PubMed Central CAS Google Scholar
146.
Mack DR, Ahrne S, Hyde L, Wei S, Hollingsworth MA. Extracellular MUC3 mucin secretion follows adherence of Lactobacillus strains to intestinal epithelial cells in vitro. Gut. 2003;52:827–33.
PubMed PubMed Central Article CAS Google Scholar
147.
Mattar AF, Teitelbaum DH, Drongowski RA, Yongyi F, Harmon CM, Coran AG. Probiotics up-regulate MUC-2 mucin gene expression in a Caco-2 cell-culture model. Pediatr Surg Int. 2002;18:586–90. https://doi.org/10.1007/s00383-002-0855-7.
Article PubMed CAS PubMed Central Google Scholar
148.
Zuhl M, Schneider S, Lanphere K, Conn C, Dokladny K, Moseley P. Exercise regulation of intestinal tight junction proteins. Br J Sports Med. 2014;48:980–6. https://doi.org/10.1136/bjsports-2012-091585.
Article PubMed PubMed Central Google Scholar
149.
Flynn MG, McFarlin BK. Toll-like receptor 4: link to the anti-inflammatory effects of exercise? Exerc Sport Sci Rev. 2006;34:176–81. https://doi.org/10.1249/01.jes.0000240027.22749.14.
Article PubMed PubMed Central Google Scholar
150.
Stuempfle KJ, Valentino T, Hew-Butler T, Hecht FM, Hoffman MD. Nausea is associated with endotoxemia during a 161-km ultramarathon. J Sports Sci. 1662-1668;2016:34. https://doi.org/10.1080/02640414.2015.1130238.
151.
Munford RS. Sensing gram-negative bacterial lipopolysaccharides: a human disease determinant? Infect Immun. 2008;76:454–65. https://doi.org/10.1128/IAI.00939-07.
Article PubMed CAS PubMed Central Google Scholar
152.
Mach N, Fuster-Botella D. Endurnace exercise and gut microbiota: a review. J Sport Health Sci. 2017;6:179–97.
PubMed Article PubMed Central Google Scholar
153.
Lescheid D. Probiotics as regulators of inflammation: a review. Functional Foods in Health and Disease. 2014;4:299–311.
154.
Pagnini C, Saeed R, Bamias G, Arseneau KO, Pizarro TT, Cominelli F. Probiotics promote gut health through stimulation of epithelial innate immunity. Proc Natl Acad Sci U S A. 2010;107:454–9. https://doi.org/10.1073/pnas.0910307107.
155.
Karczewski J, Troost FJ, Konings I, Dekker J, Kleerebezem M, Brummer RJ, Wells JM. Regulation of human epithelial tight junction proteins by Lactobacillus plantarum in vivo and protective effects on the epithelial barrier. Am J Physiol Gastrointest Liver Physiol. 2010;298:G851–9. https://doi.org/10.1152/ajpgi.00327.2009.
Article PubMed CAS Google Scholar
156.
Cario E, Gerken G, Podolsky DK. Toll-like receptor 2 controls mucosal inflammation by regulating epithelial barrier function. Gastroenterology. 2007;132:1359–74. https://doi.org/10.1053/j.gastro.2007.02.056.
Article PubMed CAS Google Scholar
157.
Perdigon G, Maldonado Galdeano C, Valdez JC, Medici M. Interaction of lactic acid bacteria with the gut immune system. Eur J Clin Nutr. 2002;56(Suppl 4):S21–6. https://doi.org/10.1038/sj.ejcn.1601658.
Article PubMed CAS Google Scholar
158.
Schiffrin EJ, Brassart D, Servin AL, Rochat F, Donnet-Hughes A. Immune modulation of blood leukocytes in humans by lactic acid bacteria: criteria for strain selection. Am J Clin Nutr. 1997;66:515S–20S. https://doi.org/10.1093/ajcn/66.2.515S.
Article PubMed CAS Google Scholar
159.
Hirano J, Yoshida T, Sugiyama T, Koide N, Mori I, Yokochi T. The effect of Lactobacillus rhamnosus on enterohemorrhagic Escherichia coli infection of human intestinal cells in vitro. Microbiol Immunol. 2003;47:405–9.
PubMed Article CAS Google Scholar
160.
Kim YS, Ho SB. Intestinal goblet cells and mucins in health and disease: recent insights and progress. Curr Gastroenterol Rep. 2010;12:319–30. https://doi.org/10.1007/s11894-010-0131-2.
Article PubMed PubMed Central Google Scholar
161.
Ayabe T, Satchell DP, Wilson CL, Parks WC, Selsted ME, Ouellette AJ. Secretion of microbicidal alpha-defensins by intestinal Paneth cells in response to bacteria. Nat Immunol. 2000;1:113–8. https://doi.org/10.1038/77783.
Article PubMed CAS Google Scholar
162.
Ostaff MJ, Stange EF, Wehkamp J. Antimicrobial peptides and gut microbiota in homeostasis and pathology. EMBO Mol Med. 2013;5:1465–83. https://doi.org/10.1002/emmm.201201773.
Article PubMed PubMed Central CAS Google Scholar
163.
Furrie E, Macfarlane S, Kennedy A, Cummings JH, Walsh SV, O’Neil DA, Macfarlane GT. Synbiotic therapy (Bifidobacterium longum/Synergy 1) initiates resolution of inflammation in patients with active ulcerative colitis: a randomised controlled pilot trial. Gut. 2005;54:242–9. https://doi.org/10.1136/gut.2004.044834.
Article PubMed PubMed Central CAS Google Scholar
164.
Servin AL. Antagonistic activities of lactobacilli and bifidobacteria against microbial pathogens. FEMS Microbiol Rev. 2004;28:405–40. https://doi.org/10.1016/j.femsre.2004.01.003.
Article PubMed CAS PubMed Central Google Scholar
165.
Chenoll E, Casinos B, Bataller E, Astals P, Echevarria J, Iglesias JR, Balbarie P, Ramon D, Genoves S. Novel probiotic Bifidobacterium bifidum CECT 7366 strain active against the pathogenic bacterium helicobacter pylori. Appl Environ Microbiol. 2011;77:1335–43. https://doi.org/10.1128/AEM.01820-10.
Article PubMed CAS PubMed Central Google Scholar
166.
Chu H, Kang S, Ha S, Cho K, Park SM, Han KH, Kang SK, Lee H, Han SH, Yun CH, et al. Lactobacillus acidophilus expressing recombinant K99 adhesive fimbriae has an inhibitory effect on adhesion of enterotoxigenic Escherichia coli. Microbiol Immunol. 2005;49:941–8.
PubMed Article CAS PubMed Central Google Scholar
167.
Munoz JA, Chenoll E, Casinos B, Bataller E, Ramon D, Genoves S, Montava R, Ribes JM, Buesa J, Fabrega J, et al. Novel probiotic Bifidobacterium longum subsp. infantis CECT 7210 strain active against rotavirus infections. Appl Environ Microbiol. 2011;77:8775–83. https://doi.org/10.1128/AEM.05548-11.
Article PubMed CAS PubMed Central Google Scholar
168.
Sgouras D, Maragkoudakis P, Petraki K, Martinez-Gonzalez B, Eriotou E, Michopoulos S, Kalantzopoulos G, Tsakalidou E, Mentis A. In vitro and in vivo inhibition of helicobacter pylori by Lactobacillus casei strain Shirota. Appl Environ Microbiol. 2004;70:518–26.
PubMed PubMed Central Article CAS Google Scholar
169.
Todoriki K, Mukai T, Sato S, Toba T. Inhibition of adhesion of food-borne pathogens to Caco-2 cells by Lactobacillus strains. J Appl Microbiol. 2001;91:154–9.
PubMed Article CAS PubMed Central Google Scholar
170.
Tsai CC, Lin PP, Hsieh YM. Three Lactobacillus strains from healthy infant stool inhibit enterotoxigenic Escherichia coli grown in vitro. Anaerobe. 2008;14:61–7. https://doi.org/10.1016/j.anaerobe.2007.11.003.
Article PubMed CAS PubMed Central Google Scholar
171.
Nakamura S, Kuda T, An C, Kanno T, Takahashi H, Kimura B. Inhibitory effects of Leuconostoc mesenteroides 1RM3 isolated from narezushi, a fermented fish with rice, on listeria monocytogenes infection to Caco-2 cells and a/J mice. Anaerobe. 2012;18:19–24. https://doi.org/10.1016/j.anaerobe.2011.11.006.
172.
Schiffrin EJ, Blum S. Interactions between the microbiota and the intestinal mucosa. Eur J Clin Nutr. 2002;56(Suppl 3):S60–4. https://doi.org/10.1038/sj.ejcn.1601489.
Article PubMed PubMed Central Google Scholar
173.
Fujiwara S, Hashiba H, Hirota T, Forstner JF. Inhibition of the binding of enterotoxigenic Escherichia coli Pb176 to human intestinal epithelial cell line HCT-8 by an extracellular protein fraction containing BIF of Bifidobacterium longum SBT2928: suggestive evidence of blocking of the binding receptor gangliotetraosylceramide on the cell surface. Int J Food Microbiol. 2001;67:97–106.
PubMed Article CAS Google Scholar
174.
Neeser JR, Granato D, Rouvet M, Servin A, Teneberg S, Karlsson KA. Lactobacillus johnsonii La1 shares carbohydrate-binding specificities with several enteropathogenic bacteria. Glycobiology. 2000;10:1193–9.
PubMed Article CAS Google Scholar
175.
Mukai T, Asasaka T, Sato E, Mori K, Matsumoto M, Ohori H. Inhibition of binding of helicobacter pylori to the glycolipid receptors by probiotic Lactobacillus reuteri. FEMS Immunol Med Microbiol. 2002;32:105–10.
PubMed Article CAS PubMed Central Google Scholar
176.
Coconnier MH, Bernet MF, Chauviere G, Servin AL. Adhering heat-killed human Lactobacillus acidophilus, strain LB, inhibits the process of pathogenicity of diarrhoeagenic bacteria in cultured human intestinal cells. J Diarrhoeal Dis Res. 1993;11:235–42.
PubMed CAS PubMed Central Google Scholar
177.
Konstantinov SR, Kuipers EJ, Peppelenbosch MP. Functional genomic analyses of the gut microbiota for CRC screening. Nat Rev Gastroenterol Hepatol. 2013;10:741–5. https://doi.org/10.1038/nrgastro.2013.178.
Article PubMed CAS PubMed Central Google Scholar
178.
Oberg TS, Steele JL, Ingham SC, Smeianov VV, Briczinski EP, Abdalla A, Broadbent JR. Intrinsic and inducible resistance to hydrogen peroxide in Bifidobacterium species. J Ind Microbiol Biotechnol. 1947-1953;2011:38. https://doi.org/10.1007/s10295-011-0983-y.
179.
Tsilingiri K, Rescigno M. Postbiotics: what else? Benefic Microbes. 2013;4:101–7. https://doi.org/10.3920/BM2012.0046.
180.
Sharma M, Shukla G. Metabiotics: one step ahead of probiotics; an insight into mechanisms involved in Anticancerous effect in colorectal Cancer. Front Microbiol. 1940;2016:7. https://doi.org/10.3389/fmicb.2016.01940.
181.
Shenderov BA. Metabiotics: novel idea or natural development of probiotic conception. Microb Ecol Health Dis. 2013;24. https://doi.org/10.3402/mehd.v24i0.20399.
182.
Canfora EE, Jocken JW, Blaak EE. Short-chain fatty acids in control of body weight and insulin sensitivity. Nat Rev Endocrinol. 2015;11:577–91. https://doi.org/10.1038/nrendo.2015.128.
Article PubMed CAS PubMed Central Google Scholar
183.
Kimura I, Ozawa K, Inoue D, Imamura T, Kimura K, Maeda T, Terasawa K, Kashihara D, Hirano K, Tani T, et al. The gut microbiota suppresses insulin-mediated fat accumulation via the short-chain fatty acid receptor GPR43. Nat Commun. 1829;2013:4. https://doi.org/10.1038/ncomms2852.
184.
den Besten G, van Eunen K, Groen AK, Venema K, Reijngoud DJ, Bakker BM. The role of short-chain fatty acids in the interplay between diet, gut microbiota, and host energy metabolism. J Lipid Res. 2013;54:2325–40. https://doi.org/10.1194/jlr.%20R036012.
185.
Kullisaar T, Zilmer M, Mikelsaar M, Vihalemm T, Annuk H, Kairane C, Kilk A. Two antioxidative lactobacilli strains as promising probiotics. Int J Food Microbiol. 2002;72:215–24.
PubMed Article CAS PubMed Central Google Scholar
186.
Lin MY, Chang FJ. Antioxidative effect of intestinal bacteria Bifidobacterium longum ATCC 15708 and Lactobacillus acidophilus ATCC 4356. Dig Dis Sci. 1617-1622;2000:45.
187.
Saide JA, Gilliland SE. Antioxidative activity of lactobacilli measured by oxygen radical absorbance capacity. J Dairy Sci. 2005;88:1352–7. https://doi.org/10.3168/jds.%20S0022-0302(05)72801-0.
Article PubMed CAS PubMed Central Google Scholar
188.
Coqueiro AY, de Oliveira Garcia AB, Rogero MM, Tirapegui J. Probiotic supplementation in sports and physical exercise: does it present any ergogenic effect? Nutr Health. 2017;23:239–49. https://doi.org/10.1177/0260106017721000.
Article PubMed CAS PubMed Central Google Scholar
189.
Akbari V, Hendijani F. Effects of probiotic supplementation in patients with type 2 diabetes: systematic review and meta-analysis. Nutr Rev. 2016;74:774–84. https://doi.org/10.1093/nutrit/nuw039.
Article PubMed PubMed Central Google Scholar
190.
Ruan Y, Sun J, He J, Chen F, Chen R, Chen H. Effect of probiotics on glycemic control: a systematic review and meta-analysis of randomized, Controlled Trials. PLoS One. 2015;10:e0132121. https://doi.org/10.1371/journal.pone.0132121.
Article PubMed PubMed Central CAS Google Scholar
191.
Alakomi HL, Skytta E, Saarela M, Mattila-Sandholm T, Latva-Kala K, Helander IM. Lactic acid permeabilizes gram-negative bacteria by disrupting the outer membrane. Appl Environ Microbiol. 2001-2005;2000:66.
192.
De Keersmaecker SC, Verhoeven TL, Desair J, Marchal K, Vanderleyden J, Nagy I. Strong antimicrobial activity of Lactobacillus rhamnosus GG against Salmonella typhimurium is due to accumulation of lactic acid. FEMS Microbiol Lett. 2006;259:89–96. https://doi.org/10.1111/j.1574-6968.2006.00250.x.
Article PubMed CAS PubMed Central Google Scholar
193.
Makras L, Triantafyllou V, Fayol-Messaoudi D, Adriany T, Zoumpopoulou G, Tsakalidou E, Servin A, De Vuyst L. Kinetic analysis of the antibacterial activity of probiotic lactobacilli towards Salmonella enterica serovar typhimurium reveals a role for lactic acid and other inhibitory compounds. Res Microbiol. 2006;157:241–7. https://doi.org/10.1016/j.resmic.2005.09.002.
Article PubMed CAS PubMed Central Google Scholar
194.
Russell JB, Diez-Gonzalez F. The effects of fermentation acids on bacterial growth. Adv Microb Physiol. 1998;39:205–34.
PubMed Article CAS PubMed Central Google Scholar
195.
Macouzet M, Lee BH, Robert N. Production of conjugated linoleic acid by probiotic Lactobacillus acidophilus La-5. J Appl Microbiol. 1886-1891;2009:106. https://doi.org/10.1111/j.1365-2672.2009.04164.x.
196.
O’Shea EF, Cotter PD, Stanton C, Ross RP, Hill C. Production of bioactive substances by intestinal bacteria as a basis for explaining probiotic mechanisms: bacteriocins and conjugated linoleic acid. Int J Food Microbiol. 2012;152:189–205. https://doi.org/10.1016/j.ijfoodmicro.2011.05.025.
Article PubMed CAS PubMed Central Google Scholar
197.
Lee K, Paek K, Lee HY, Park JH, Lee Y. Antiobesity effect of trans-10,cis-12-conjugated linoleic acid-producing Lactobacillus plantarum PL62 on diet-induced obese mice. J Appl Microbiol. 2007;103:1140–6. https://doi.org/10.1111/j.1365-2672.2007.03336.x.
Article PubMed CAS PubMed Central Google Scholar
198.
Nieman DC, Henson DA, Austin MD, Sha W. Upper respiratory tract infection is reduced in physically fit and active adults. Br J Sports Med. 2011;45:987–92. https://doi.org/10.1136/bjsm.2010.077875.
Article PubMed PubMed Central Google Scholar
199.
Gleeson M, Bishop NC. URI in athletes: are mucosal immunity and cytokine responses key risk factors? Exerc Sport Sci Rev. 2013;41:148–53. https://doi.org/10.1097/JES.0b013e3182956ead.
Article PubMed PubMed Central Google Scholar
200.
Walsh NP. Recommendations to maintain immune health in athletes. Eur J Sport Sci. 2018;18:820–31. https://doi.org/10.1080/17461391.2018.1449895.
Article PubMed PubMed Central Google Scholar
201.
Walsh NP, Gleeson M, Shephard RJ, Gleeson M, Woods JA, Bishop NC, Fleshner M, Green C, Pedersen BK, Hoffman-Goetz L, et al. Position statement. Part one: Immune function and exercise. Exerc Immunol Rev. 2011;17:6–63.
PubMed PubMed Central Google Scholar
202.
Gomez-Llorente C, Munoz S, Gil A. Role of toll-like receptors in the development of immunotolerance mediated by probiotics. Proc Nutr Soc. 2010;69:381–9. https://doi.org/10.1017/S0029665110001527.
Article PubMed CAS PubMed Central Google Scholar
203.
Lebeer S, Vanderleyden J, De Keersmaecker SC. Host interactions of probiotic bacterial surface molecules: comparison with commensals and pathogens. Nat Rev Microbiol. 2010;8:171–84. https://doi.org/10.1038/nrmicro2297.
Article PubMed CAS PubMed Central Google Scholar
204.
Bermon S, Petriz B, Kajeniene A, Prestes J, Castell L, Franco OL. The microbiota: an exercise immunology perspective. Exerc Immunol Rev. 2015;21:70–9.
PubMed PubMed Central Google Scholar
205.
Lancaster GI, Halson SL, Khan Q, Drysdale P, Wallace F, Jeukendrup AE, Drayson MT, Gleeson M. Effects of acute exhaustive exercise and chronic exercise training on type 1 and type 2 T lymphocytes. Exerc Immunol Rev. 2004;10:91–106.
PubMed PubMed Central Google Scholar
206.
Martin SA, Pence BD, Woods JA. Exercise and respiratory tract viral infections. Exerc Sport Sci Rev. 2009;37:157–64. https://doi.org/10.1097/JES.0b013e3181b7b57b.
Article PubMed PubMed Central Google Scholar
207.
Jager R, Purpura M, Farmer S, Cash HA, Keller D. Probiotic Bacillus coagulans GBI-30, 6086 improves protein absorption and utilization. Probiotics Antimicrob Proteins. 2018;10:611–5. https://doi.org/10.1007/s12602-017-9354-y.
Article PubMed CAS PubMed Central Google Scholar
208.
Keller D, Van Dinter R, Cash H, Farmer S, Venema K. Bacillus coagulans GBI-30, 6086 increases plant protein digestion in a dynamic, computer-controlled in vitro model of the small intestine (TIM-1). Benefic Microbes. 2017;8:491–6. https://doi.org/10.3920/BM2016.0196.
209.
Buckley JD, Thomson RL, Coates AM, Howe PR, DeNichilo MO, Rowney MK. Supplementation with a whey protein hydrolysate enhances recovery of muscle force-generating capacity following eccentric exercise. J Sci Med Sport. 2010;13:178–81. https://doi.org/10.1016/j.jsams.2008.06.007.
Article PubMed PubMed Central Google Scholar
210.
Benton D, Williams C, Brown A. Impact of consuming a milk drink containing a probiotic on mood and cognition. Eur J Clin Nutr. 2007;61:355–61. https://doi.org/10.1038/sj.ejcn.1602546.
Article PubMed CAS PubMed Central Google Scholar
211.
de Simone C. The unregulated probiotic market. Clin Gastroenterol Hepatol. 2018. https://doi.org/10.1016/j.cgh.2018.01.018.
PubMed Article PubMed Central Google Scholar
212.
World Gastroenterology Organisation. Global Guidelines: Probiotics and Prebiotics. World Gastroentereology Oganisation. Milwaukee; 2017.
213.
Sanders ME, Akkermans LM, Haller D, Hammerman C, Heimbach J, Hormannsperger G, Huys G, Levy DD, Lutgendorff F, Mack D, et al. Safety assessment of probiotics for human use. Gut Microbes. 2010;1:164–85. https://doi.org/10.4161/gmic.1.3.12127.
Article PubMed PubMed Central Google Scholar
214.
Marteau P. Safety aspects of probiotic products. Naringsforskning. 2001;45:22–4.
215.
Cesaro C, Tiso A, Del Prete A, Cariello R, Tuccillo C, Cotticelli G, Del Vecchio Blanco C, Loguercio C. Gut microbiota and probiotics in chronic liver diseases. Dig Liver Dis. 2011;43:431–8. https://doi.org/10.1016/j.dld.2010.10.015.
Article PubMed PubMed Central Google Scholar
216.
Besselink MG, van Santvoort HC, Buskens E, Boermeester MA, van Goor H, Timmerman HM, Nieuwenhuijs VB, Bollen TL, van Ramshorst B, Witteman BJ, et al. Probiotic prophylaxis in predicted severe acute pancreatitis: a randomised, double-blind, placebo-controlled trial. Lancet. 2008;371:651–9. https://doi.org/10.1016/S0140-6736(08)60207-X.
Article PubMed PubMed Central Google Scholar
217.
Sanders ME, Merenstein DJ, Ouwehand AC, Reid G, Salminen S, Cabana MD, Paraskevakos G, Leyer G. Probiotic use in at-risk populations. J Am Pharm Assoc (2003). 2016;56:680–6. https://doi.org/10.1016/j.japh.2016.07.001.
218.
Vallabhaneni S, Walker TA, Lockhart SR, Ng D, Chiller T, Melchreit R, Brandt ME, Smith RM. Centers for Disease Control and Prevention, see https://www.cdc.gov/mmwr/preview/mmwrhtml/mm6406a6.htm.
219.
FDA. New Dietary Ingredients Notification Process. Washington; 2016.
220.
Didari T, Solki S, Mozaffari S, Nikfar S, Abdollahi M. A systematic review of the safety of probiotics. Expert Opin Drug Saf. 2014;13:227–39. https://doi.org/10.1517/14740338.2014.872627.
Article PubMed PubMed Central Google Scholar
221.
Boyle RJ, Robins-Browne RM, Tang ML. Probiotic use in clinical practice: what are the risks? Am J Clin Nutr. 2006;83:1256–64; quiz 1446-1257. https://doi.org/10.1093/ajcn/83.6.1256.
Article PubMed CAS PubMed Central Google Scholar
222.
Tapiovaara L, Lehtoranta L, Poussa T, Makivuokko H, Korpela R, Pitkaranta A. Absence of adverse events in healthy individuals using probiotics–analysis of six randomised studies by one study group. Benefic Microbes. 2016;7:161–9. https://doi.org/10.3920/BM2015.0096.
223.
Larsen CN, Nielsen S, Kaestel P, Brockmann E, Bennedsen M, Christensen HR, Eskesen DC, Jacobsen BL, Michaelsen KF. Dose-response study of probiotic bacteria Bifidobacterium animalis subsp lactis BB-12 and Lactobacillus paracasei subsp paracasei CRL-341 in healthy young adults. Eur J Clin Nutr. 2006;60:1284–93. https://doi.org/10.1038/sj.ejcn.1602450.
Article PubMed CAS PubMed Central Google Scholar
224.
Ford AC, Quigley EM, Lacy BE, Lembo AJ, Saito YA, Schiller LR, Soffer EE, Spiegel BM, Moayyedi P. Efficacy of prebiotics, probiotics, and synbiotics in irritable bowel syndrome and chronic idiopathic constipation: systematic review and meta-analysis. Am J Gastroenterol, 2014;109:1547–61; quiz 1546, 1562. https://doi.org/10.1038/ajg.2014.202.
PubMed Article PubMed Central Google Scholar
225.
Monteiro R, Azevedo I. Chronic inflammation in obesity and the metabolic syndrome. Mediat Inflamm. 2010;2010. https://doi.org/10.1155/2010/289645.
226.
Food and Agriculture Organization of the United Nations and World Health Organization. Joint FAO/WHO working group report on drafting guidelines fro the evaluation of probiotics in food. 2002.
227.
Health Canada. Natural Health Products Ingredients Database: Probiotics. Availabe online: http://webprod.hc-sc.gc.ca/nhpid-bdipsn/atReq.do?atid=probio&lang=eng (accessed on Oct 23).
228.
Zorzela L, Ardestani SK, McFarland LV, Vohra S. Is there a role for modified probiotics as beneficial microbes: a systematic review of the literature. Benefic Microbes. 2017;8:739–54. https://doi.org/10.3920/BM2017.0032.
229.
Health Canada. Licensed Natural Health Products Database. 2019. . Availabe online: https://health-products.canada.ca/lnhpd-bdpsnh/index-eng.jsp (accessed on Oct 23).
230.
Arora M, Baldi A. Regulatory categories of probiotics across the globe: a review representing existing and recommended categorization. Indian J Med Microbiol. 2015;33(Suppl):2–10. https://doi.org/10.4103/0255-0857.150868.
Article PubMed PubMed Central Google Scholar
231.
Amagase H. Current marketplace for probiotics: a Japanese perspective. Clin Infect Dis. 2008;46(Suppl 2):S73–5; discussion S144–151. https://doi.org/10.1086/523338.
Article PubMed PubMed Central Google Scholar
232.
European Food Standards Agency. Qualified Presumption of Safety. Available from: http://www.efsa.europa.eu/en/topics/topic/qualified-presumption-safety-qps. Access date: 10 Aug 2018.
233.
Kolacek S, Hojsak I, Berni Canani R, Guarino A, Indrio F, Orel R, Pot B, Shamir R, Szajewska H, Vandenplas Y, et al. Commercial probiotic products: a call for improved quality control. A position paper by the ESPGHAN working Group for Probiotics and Prebiotics. J Pediatr Gastroenterol Nutr. 2017;65:117–24. https://doi.org/10.1097/MPG.0000000000001603.
Article PubMed PubMed Central Google Scholar
234.
NIH. Probiotics: In Depth. https://nccih.nih.gov/health/probiotics/introduction.htm. 2016.
235.
Giordano-Schaefer, J.; Ruthsatz, M.; Schneider, H. Overcoming distinctive regulatory barriers for the development of medical foods. Availabe online: https://www.raps.org/regulatory-focus%E2%84%A2/news-articles/2017/10/overcoming-distinctive-regulatory-barriers-for-the-development-of-medical-foods (Accessed on Oct 2017).
236.
Kullen MJ, Amann MM, O’Shaughnessy MJ, O’Sullivan DJ, Busta FF, Brady LJ. Differentiation of ingested and endogenous bifidobacteria by DNA fingerprinting demonstrates the survival of an unmodified strain in the gastrointestinal tract of humans. J Nutr. 1997;127:89–94. https://doi.org/10.1093/jn/127.1.89.
Article PubMed CAS PubMed Central Google Scholar
237.
Poutahidis T, Springer A, Levkovich T, Qi P, Varian BJ, Lakritz JR, Ibrahim YM, Chatzigiagkos A, Alm EJ, Erdman SE. Probiotic microbes sustain youthful serum testosterone levels and testicular size in aging mice. PLoS One. 2014;9:e84877. https://doi.org/10.1371/journal.pone.0084877.
Article PubMed PubMed Central CAS Google Scholar
238.
Lyte M. Probiotics function mechanistically as delivery vehicles for neuroactive compounds: microbial endocrinology in the design and use of probiotics. Bioessays. 2011;33:574–81. https://doi.org/10.1002/bies.201100024.
Article PubMed CAS PubMed Central Google Scholar
239.
Bravo JA, Forsythe P, Chew MV, Escaravage E, Savignac HM, Dinan TG, Bienenstock J, Cryan JF. Ingestion of Lactobacillus strain regulates emotional behavior and central GABA receptor expression in a mouse via the vagus nerve. Proc Natl Acad Sci U S A. 2011;108:16050–5. https://doi.org/10.1073/pnas.1102999108.
Article PubMed PubMed Central Google Scholar
240.
Steenbergen L, Sellaro R, van Hemert S, Bosch JA, Colzato LS. A randomized controlled trial to test the effect of multispecies probiotics on cognitive reactivity to sad mood. Brain Behav Immun. 2015;48:258–64. https://doi.org/10.1016/j.bbi.2015.04.003.
Article PubMed PubMed Central Google Scholar
241.
Kim J, Yun JM, Kim MK, Kwon O, Cho B. Lactobacillus gasseri BNR17 supplementation reduces the visceral fat accumulation and waist circumference in obese adults: a randomized, double-blind, Placebo-Controlled Trial. J Med Food. 2018;21:454–61. https://doi.org/10.1089/jmf.2017.3937.
Article PubMed CAS PubMed Central Google Scholar
242.
Sanchez M, Darimont C, Drapeau V, Emady-Azar S, Lepage M, Rezzonico E, Ngom-Bru C, Berger B, Philippe L, Ammon-Zuffrey C, et al. Effect of Lactobacillus rhamnosus CGMCC1.3724 supplementation on weight loss and maintenance in obese men and women. Br J Nutr. 2014;111:1507–19. https://doi.org/10.1017/S0007114513003875.
Article PubMed CAS PubMed Central Google Scholar
243.
Gauchard GC, Gangloff P, Vouriot A, Mallie JP, Perrin PP. Effects of exercise-induced fatigue with and without hydration on static postural control in adult human subjects. Int J Neurosci. 2002;112:1191–206.
PubMed Article PubMed Central Google Scholar
244.
Green H, Halestrap A, Mockett C, O’Toole D, Grant S, Ouyang J. Increases in muscle MCT are associated with reductions in muscle lactate after a single exercise session in humans. Am J Physiol Endocrinol Metab. 2002;282:E154–60. https://doi.org/10.1152/ajpendo.2002.282.1.E154.
Article PubMed CAS PubMed Central Google Scholar
245.
Hobson RM, Saunders B, Ball G, Harris RC, Sale C. Effects of beta-alanine supplementation on exercise performance: a meta-analysis. Amino Acids. 2012;43:25–37. https://doi.org/10.1007/s00726-011-1200-z.
Article PubMed PubMed Central CAS Google Scholar
246.
Brooks SP, Storey KB. A quantitative evaluation of the effect of enzyme complexes on the glycolytic rate in vivo: mathematical modeling of the glycolytic complex. J Theor Biol. 1991;149:361–75.
PubMed Article CAS PubMed Central Google Scholar
247.
Duncan SH, Louis P, Flint HJ. Lactate-utilizing bacteria, isolated from human feces, that produce butyrate as a major fermentation product. Appl Environ Microbiol. 2004;70:5810–7. https://doi.org/10.1128/AEM.70.10.5810-5817.2004.
Article PubMed PubMed Central CAS Google Scholar
248.
Hsu YJ, Huang WC, Lin JS, Chen YM, Ho ST, Huang CC, Tung YT. Kefir supplementation modifies gut microbiota composition, reduces physical fatigue, and improves exercise performance in mice. Nutrients. 2018;10. https://doi.org/10.3390/nu10070862.
PubMed Central Article CAS Google Scholar
249.
ANVISA-Ministry of Health. Technical Regulation of Bioeactive and Probiotic Substances Isolated with Allegations of Functional or Health Properties. 2008.
250.
Ministerio della Salute. Linee guida su probiotici e prebioticic. Available oneline at: http://www.salute.gov; 2013.
251.
Al-Asmakh M, Stukenborg JB, Reda A, Anuar F, Strand ML, Hedin L, Pettersson S, Soder O. The gut microbiota and developmental programming of the testis in mice. PLoS One. 2014;9:e103809. https://doi.org/10.1371/journal.pone.0103809.
Article PubMed PubMed Central CAS Google Scholar
252.
Harada N, Hanaoka R, Horiuchi H, Kitakaze T, Mitani T, Inui H, Yamaji R. Castration influences intestinal microflora and induces abdominal obesity in high-fat diet-fed mice. Sci Rep. 2016;6:23001. https://doi.org/10.1038/srep23001.
Article PubMed PubMed Central CAS Google Scholar
253.
Ibrahim HA, Zhu Y, Wu C, Lu C, Ezekwe MO, Liao SF, Huang K. Selenium-enriched probiotics improves murine male fertility compromised by high fat diet. Biol Trace Elem Res. 2012;147:251–60. https://doi.org/10.1007/s12011-011-9308-2.
Article PubMed CAS PubMed Central Google Scholar
254.
Lee J, Yang W, Hostetler A, Schultz N, Suckow MA, Stewart KL, Kim DD, Kim HS. Characterization of the anti-inflammatory Lactobacillus reuteri BM36301 and its probiotic benefits on aged mice. BMC Microbiol. 2016;16:69. https://doi.org/10.1186/s12866-016-0686-7.
Article PubMed PubMed Central CAS Google Scholar
255.
McMullen MH, Hamilton-Reeves JM, Bonorden MJ, Wangen KE, Phipps WR, Feirtag JM, Kurzer MS. Consumption of Lactobacillus acidophilus and Bifidobacterium longum does not alter phytoestrogen metabolism and plasma hormones in men: a pilot study. J Altern Complement Med. 2006;12:887–94. https://doi.org/10.1089/acm.2006.12.887.
Article PubMed PubMed Central Google Scholar
256.
Maretti C, Cavallini G. The association of a probiotic with a prebiotic (Flortec, Bracco) to improve the quality/quantity of spermatozoa in infertile patients with idiopathic oligoasthenoteratospermia: a pilot study. Andrology. 2017;5:439–44. https://doi.org/10.1111/andr.12336.
Article PubMed CAS PubMed Central Google Scholar
257.
Tremellen K. Gut endotoxin leading to a decline IN gonadal function (GELDING) – a novel theory for the development of late onset hypogonadism in obese men. Basic Clin Androl. 2016;26:7. https://doi.org/10.1186/s12610-016-0034-7.
Article PubMed PubMed Central Google Scholar
258.
Tremellen K, McPhee N, Pearce K. Metabolic endotoxaemia related inflammation is associated with hypogonadism in overweight men. Basic Clin Androl. 2017;27:5. https://doi.org/10.1186/s12610-017-0049-8.
Article PubMed PubMed Central Google Scholar
259.
Tremellen K, McPhee N, Pearce K, Benson S, Schedlowski M, Engler H. Endotoxin-initiated inflammation reduces testosterone production in men of reproductive age. Am J Physiol Endocrinol Metab. 2018;314:E206–13. https://doi.org/10.1152/ajpendo.00279.2017.
Article PubMed CAS PubMed Central Google Scholar
260.
De Lorenzo A, Noce A, Moriconi E, Rampello T, Marrone G, Di Daniele N, Rovella V. MOSH syndrome (male obesity secondary hypogonadism): clinical assessment and possible therapeutic approaches. Nutrients. 2018;10. https://doi.org/10.3390/nu10040474.
PubMed Central Article CAS Google Scholar
261.
Pique N, Berlanga M, Minana-Galbis D. Health benefits of heat-killed (Tyndallized) probiotics: An overview. Int J Mol Sci. 2019;20(10):2534. https://doi.org/10.3390/ijms20102534.
PubMed Central Article Google Scholar
262.
Komano Y, Shimada K, Naito H, Fukao K, Ishihara Y, Fujii T, Kokubo T, Daida H. Efficacy of heat-killed Lactococcus lactis JCM 5805 on immunity and fatigue during consecutive high intensity exercise in male athletes: a randomized, placebo-controlled, double-blinded trial. J Int Soc Sports Nutr. 2018;15:39. https://doi.org/10.1186/s12970-018-0244-9.
Article PubMed PubMed Central CAS Google Scholar
263.
Kalman D, Hewlings S. Inactivated probiotic Bacillus coagulans GBI-30 demonstrates Immunosupportive properties in healthy adults following stressful exercise. J Probiotics and Health. 2018;6.
264.
Taverniti V, Guglielmetti S. The immunomodulatory properties of probiotic microorganisms beyond their viability (ghost probiotics: proposal of paraprobiotic concept). Genes Nutr. 2011;6:261–74. https://doi.org/10.1007/s12263-011-0218-x.
Article PubMed PubMed Central CAS Google Scholar
265.
Deshpande G, Athalye-Jape G, Patole S. Para-probiotics for preterm neonates-the next frontier. Nutrients. 2018;10. https://doi.org/10.3390/nu10070871.
PubMed Central Article CAS Google Scholar
266.
Sugahara H, Yao R, Odamaki T, Xiao JZ. Differences between live and heat-killed bifidobacteria in the regulation of immune function and the intestinal environment. Benefic Microbes. 2017;8:463–72. https://doi.org/10.3920/BM2016.0158.
267.
Burta O, Iacobescu C, Mateescu RB, Nicolaie T, Tiuca N, Pop CS. Efficacy and safety of APT036 versus simethicone in the treatment of functional bloating: a multicentre, randomised, double-blind, parallel group, clinical study. Transl Gastroenterol Hepatol. 2018;3, 72. https://doi.org/10.21037/tgh.2018.09.11.
PubMed PubMed Central Article Google Scholar
268.
Vandenplas Y, Bacarea A, Marusteri M, Bacarea V, Constantin M, Manolache M. Efficacy and safety of APT198K for the treatment of infantile colic: a pilot study. J Comp Eff Res. 2017;6:137–44. https://doi.org/10.2217/cer-2016-0059.
Article PubMed PubMed Central Google Scholar
269.
Hirose Y, Yamamoto Y, Yoshikai Y, Murosaki S. Oral intake of heat-killed Lactobacillus plantarum L-137 decreases the incidence of upper respiratory tract infection in healthy subjects with high levels of psychological stress. J Nutr Sci. 2013;2:e39. https://doi.org/10.1017/jns.2013.35.
Article PubMed PubMed Central CAS Google Scholar
270.
Arimori Y, Nakamura R, Hirose Y, Murosaki S, Yamamoto Y, Shidara O, Ichikawa H, Yoshikai Y. Daily intake of heat-killed Lactobacillus plantarum L-137 enhances type I interferon production in healthy humans and pigs. Immunopharmacol Immunotoxicol. 2012;34(6):937–43. https://doi.org/10.3109/08923973.2012.672425.
PubMed Article CAS PubMed Central Google Scholar
271.
Sawada D, Kuwano Y, Tanaka T, Hara S, Uchiyama Y, Sugawara T, Fujiwara S, Rokutan K, Nishida K. Daily intake of Lactobacillus gasseri CP2305 relieves fatigue and stress-related symptoms in male university Ekiden runners: A double-blind, randomized, and placebo-controlled clinical trial. J Funct Foods. 2019;57:465–76.
272.
Nishida K, Sawadab D, Kuwanoa Y, Tanakaa H, Sugawarab T, Aokib Y, Fujiwarab S, Rokutana K. Daily administration of paraprobiotic Lactobacillus gasseri CP2305 ameliorates chronic stress-associated symptoms in Japanese medical students. J Funct Foods. 2017;36:112–21.
273.
Friedrich MJ. Depression is the leading cause of disability around the world. JAMA. 2017;317:1517. https://doi.org/10.1001/jama.2017.3826.
Article PubMed PubMed Central Google Scholar
274.
Maes M, Kubera M, Leunis JC, Berk M, Geffard M, Bosmans E. In depression, bacterial translocation may drive inflammatory responses, oxidative and nitrosative stress (O&NS), and autoimmune responses directed against O&NS-damaged neoepitopes. Acta Psychiatr Scand. 2013;127:344–54. https://doi.org/10.1111/j.1600-0447.2012.01908.x.
Article PubMed CAS Google Scholar
275.
Clarke G, Grenham S, Scully P, Fitzgerald P, Moloney RD, Shanahan F, Dinan TG, Cryan JF. The microbiome-gut-brain axis during early life regulates the hippocampal serotonergic system in a sex-dependent manner. Mol Psychiatry. 2013;18:666–73. https://doi.org/10.1038/mp.2012.77.
Article PubMed CAS Google Scholar
276.
Vitetta L, Bambling M, Alford H. The gastrointestinal tract microbiome, probiotics, and mood. Inflammopharmacology. 2014;22:333–9. https://doi.org/10.1007/s10787-014-0216-x.
Article PubMed CAS Google Scholar
277.
Rao AV, Bested AC, Beaulne TM, Katzman MA, Iorio C, Berardi JM, Logan AC. A randomized, double-blind, placebo-controlled pilot study of a probiotic in emotional symptoms of chronic fatigue syndrome. Gut Pathog. 2009;1:6. https://doi.org/10.1186/1757-4749-1-6.
Article PubMed PubMed Central Google Scholar
278.
Messaoudi M, Lalonde R, Violle N, Javelot H, Desor D, Nejdi A, Bisson JF, Rougeot C, Pichelin M, Cazaubiel M, et al. Assessment of psychotropic-like properties of a probiotic formulation (Lactobacillus helveticus R0052 and Bifidobacterium longum R0175) in rats and human subjects. Br J Nutr. 2011;105:755–64. https://doi.org/10.1017/S0007114510004319.
Article PubMed CAS Google Scholar
279.
Marotta A, Sarno E, Del Casale A, Pane M, Mogna L, Amoruso A, Felis GE, Fiorio M. Effects of probiotics on cognitive reactivity, mood, and sleep quality. Front Psychiatry. 2019;10:164. https://doi.org/10.3389/fpsyt.2019.00164.
Article PubMed PubMed Central Google Scholar
280.
Clark A, Mach N. Exercise-induced stress behavior, gut-microbiota-brain axis and diet: a systematic review for athletes. J Int Soc Sports Nutr. 2016;13:43. https://doi.org/10.1186/s12970-016-0155-6.
Article PubMed PubMed Central CAS Google Scholar
281.
Zhao S, Liu W, Wang J, Shi J, Sun Y, Wang W, Ning G, Liu R, Hong J. Akkermansia muciniphila improves metabolic profiles by reducing inflammation in chow diet-fed mice. J Mol Endocrinol. 2017;58:1–14. https://doi.org/10.1530/JME-16-0054.
282.
Warber JP, Patton JF, Tharion WJ, Zeisel SH, Mello RP, Kemnitz CP, Lieberman HR. The effects of choline supplementation on physical performance. Int J Sport Nutr Exerc Metab. 2000;10:170–81.
PubMed Article CAS Google Scholar
283.
Martin FP, Wang Y, Sprenger N, Holmes E, Lindon JC, Kochhar S, Nicholson JK. Effects of probiotic Lactobacillus paracasei treatment on the host gut tissue metabolic profiles probed via magic-angle-spinning NMR spectroscopy. J Proteome Res. 2007;6:1471–81. https://doi.org/10.1021/pr060596a.
Article PubMed CAS Google Scholar
284.
Wang Z, Klipfell E, Bennett BJ, Koeth R, Levison BS, Dugar B, Feldstein AE, Britt EB, Fu X, Chung YM, et al. Gut flora metabolism of phosphatidylcholine promotes cardiovascular disease. Nature. 2011;472:57–63. https://doi.org/10.1038/nature09922.
Article PubMed PubMed Central CAS Google Scholar
285.
Boutagy NE, Neilson AP, Osterberg KL, Smithson AT, Englund TR, Davy BM, Hulver MW, Davy KP. Probiotic supplementation and trimethylamine-N-oxide production following a high-fat diet. Obesity (Silver Spring). 2015;23:2357–63. https://doi.org/10.1002/oby.21212.
286.
Borges NA, Stenvinkel P, Bergman P, Qureshi AR, Lindholm B, Moraes C, Stockler-Pinto MB, Mafra D. Effects of probiotic supplementation on trimethylamine-N-oxide plasma levels in hemodialysis patients: a pilot study. Probiotics Antimicrob Proteins. 2018. https://doi.org/10.1007/s12602-018-9411-1, https://doi.org/10.1007/s12602-018-9411-1.
287.
Tompkins TA, Mainville I, Arcand Y. The impact of meals on a probiotic during transit through a model of the human upper gastrointestinal tract. Benefic Microbes. 2011;2:295–303. https://doi.org/10.3920/BM2011.0022.
288.
He CS, Tsai ML, Ko MH, Chang CK, Fang SH. Relationships among salivary immunoglobulin a, lactoferrin and cortisol in basketball players during a basketball season. Eur J Appl Physiol. 2010;110:989–95. https://doi.org/10.1007/s00421-010-1574-8.
Article PubMed CAS PubMed Central Google Scholar
289.
Li TL, Lin HC, Ko MH, Chang CK, Fang SH. Effects of prolonged intensive training on the resting levels of salivary immunoglobulin a and cortisol in adolescent volleyball players. J Sports Med Phys Fitness. 2012;52:569–73.
PubMed CAS PubMed Central Google Scholar
290.
Tsai ML, Li TL, Chou LW, Chang CK, Huang SY, Fang SH. Resting salivary levels of IgA and cortisol are significantly affected during intensive resistance training periods in elite male weightlifters. J Strength Cond Res. 2012;26:2202–8. https://doi.org/10.1519/JSC.0b013e31823a4246.
Article PubMed PubMed Central Google Scholar
291.
Ait-Belgnaoui A, Durand H, Cartier C, Chaumaz G, Eutamene H, Ferrier L, Houdeau E, Fioramonti J, Bueno L, Theodorou V. Prevention of gut leakiness by a probiotic treatment leads to attenuated HPA response to an acute psychological stress in rats. Psychoneuroendocrinology. 2012;37:1885–95. https://doi.org/10.1016/j.psyneuen.2012.03.024.
Article PubMed CAS PubMed Central Google Scholar
292.
Knowles SR, Nelson EA, Palombo EA. Investigating the role of perceived stress on bacterial flora activity and salivary cortisol secretion: a possible mechanism underlying susceptibility to illness. Biol Psychol. 2008;77:132–7. https://doi.org/10.1016/j.biopsycho.2007.09.010.
Article PubMed PubMed Central Google Scholar
293.
Andersson H, Tullberg C, Ahrne S, Hamberg K, Lazou Ahren I, Molin G, Sonesson M, Hakansson A. Oral Administration of Lactobacillus plantarum 299v reduces cortisol levels in human saliva during examination induced stress: a randomized, Double-Blind Controlled Trial. Int J Microbiol. 2016;2016:8469018. https://doi.org/10.1155/2016/8469018.
Article PubMed PubMed Central CAS Google Scholar
294.
Kato-Kataoka A, Nishida K, Takada M, Kawai M, Kikuchi-Hayakawa H, Suda K, Ishikawa H, Gondo Y, Shimizu K, Matsuki T, et al. Fermented Milk containing Lactobacillus casei strain Shirota preserves the diversity of the gut microbiota and relieves abdominal dysfunction in healthy medical students exposed to academic stress. Appl Environ Microbiol. 2016;82:3649–58. https://doi.org/10.1128/AEM.04134-15.
Article PubMed PubMed Central CAS Google Scholar
295.
Takada M, Nishida K, Kataoka-Kato A, Gondo Y, Ishikawa H, Suda K, Kawai M, Hoshi R, Watanabe O, Igarashi T, et al. Probiotic Lactobacillus casei strain Shirota relieves stress-associated symptoms by modulating the gut-brain interaction in human and animal models. Neurogastroenterol Motil. 2016;28:1027–36. https://doi.org/10.1111/nmo.12804.
Article PubMed CAS Google Scholar
296.
Kelly JR, Allen AP, Temko A, Hutch W, Kennedy PJ, Farid N, Murphy E, Boylan G, Bienenstock J, Cryan JF, et al. Lost in translation? The potential psychobiotic Lactobacillus rhamnosus (JB-1) fails to modulate stress or cognitive performance in healthy male subjects. Brain Behav Immun. 2017;61:50–9. https://doi.org/10.1016/j.bbi.2016.11.018.
Article PubMed CAS PubMed Central Google Scholar
297.
Reis DJ, Ilardi SS, Punt SEW. The anxiolytic effect of probiotics: a systematic review and meta-analysis of the clinical and preclinical literature. PLoS One. 2018;13:e0199041. https://doi.org/10.1371/journal.pone.0199041.
Article PubMed PubMed Central CAS Google Scholar
298.
Desbrow B, McCormack J, Burke LM, Cox GR, Fallon K, Hislop M, Logan R, Marino N, Sawyer SM, Shaw G, et al. Sports dietitians Australia position statement: sports nutrition for the adolescent athlete. Int J Sport Nutr Exerc Metab. 2014;24:570–84. https://doi.org/10.1123/ijsnem.2014-0031.
Article PubMed CAS PubMed Central Google Scholar
299.
Shaw MT, Leggat PA, Chatterjee S. Travelling to India for the Delhi XIX commonwealth games 2010. Travel Med Infect Dis. 2010;8:129–38. https://doi.org/10.1016/j.tmaid.2010.04.007.